Coastal Vegetated Ecosystems: Mangrove Forests, Salt Marshes, and Seagrass Beds
Overview
Mangrove forests, salt marshes, and seagrass beds grow where land meets the sea and store carbon in their vegetation and underlying sediments/soils. The sediments/soils can store both organic and inorganic carbon. Some of this carbon originates from within the ecosystem itself (autochthonous carbon) while some originate from outside the ecosystem (allochthonous carbon). The exact proportion of autochthonous versus allochthonous carbon is difficult to determine, and differs by site, but is important when trying to account for all the carbon fluxes in a system. What’s more, carbon can move laterally and vertically in, out, and through these systems, creating additional complexity for carbon accounting.
Of these three ecosystems, in terms of current global extent, current carbon storage, and carbon sequestration potential, mangrove forests are the best understood followed by salt marshes. Seagrass beds are the least well understood both in spatial extent and ultimate carbon sequestration potential.
Restoration and its limitations: CDR pathways within coastal vegetated ecosystems are primarily concerned with restoration – the act of bringing an ecosystem back as nearly as possible to its original condition[1]As defined by the US National Research Council (1992) . However, the goal of restoration may be unattainable for several reasons, including irreversible land and hydrological (starved of freshwater input) change, conditions that do not permit rehabilitation, and the inherent spatial limitations for ecosystems that occupy specific niches (Adam, 2019). Another challenge is the host of known and unknown consequences of climate change which may impact the feasibility and efficacy of restoration efforts. Monitoring of restoration projects will also be critical both as important data points for studies and predictive science as well as for any involvement with carbon markets. There has been historical reluctance from both government agencies and private developers to fund long-term monitoring(Adam, 2019), however, this will be crucial for informed decision-making about where and how to implement restoration projects.
Net sequestration of CO2e and its storage durability are key metrics when considering restoration projects for CDR. Ideally, monitoring of restoration projects should feed back into adaptive management practices, where management techniques are adjusted through an iterative process in response to other variables such as climate change.
Mangrove forests, salt marshes, and seagrass beds grow where land meets the sea and store carbon in their vegetation and underlying sediments/soils. The sediments/soils can store both organic and inorganic carbon. Some of this carbon originates from within the ecosystem itself (autochthonous carbon) while some originate from outside the ecosystem (allochthonous carbon). The exact proportion of autochthonous versus allochthonous carbon is difficult to determine, and differs by site, but is important when trying to account for all the carbon fluxes in a system. What’s more, carbon can move laterally and vertically in, out, and through these systems, creating additional complexity for carbon accounting.
Of these three ecosystems, in terms of current global extent, current carbon storage, and carbon sequestration potential, mangrove forests are the best understood followed by salt marshes. Seagrass beds are the least well understood both in spatial extent and ultimate carbon sequestration potential.
Restoration and its limitations: CDR pathways within coastal vegetated ecosystems are primarily concerned with restoration – the act of bringing an ecosystem back as nearly as possible to its original condition[1]As defined by the US National Research Council (1992) . However, the goal of restoration may be unattainable for several reasons, including irreversible land and hydrological (starved of freshwater input) change, conditions that do not permit rehabilitation, and the inherent spatial limitations for ecosystems that occupy specific niches (Adam, 2019). Another challenge is the host of known and unknown consequences of climate change which may impact the feasibility and efficacy of restoration efforts. Monitoring of restoration projects will also be critical both as important data points for studies and predictive science as well as for any involvement with carbon markets. There has been historical reluctance from both government agencies and private developers to fund long-term monitoring(Adam, 2019), however, this will be crucial for informed decision-making about where and how to implement restoration projects.
Net sequestration of CO2e and its storage durability are key metrics when considering restoration projects for CDR. Ideally, monitoring of restoration projects should feed back into adaptive management practices, where management techniques are adjusted through an iterative process in response to other variables such as climate change.
Mangrove forests, salt marshes, and seagrass beds grow where land meets the sea and store carbon in their vegetation and underlying sediments/soils. The sediments/soils can store both organic and inorganic carbon. Some of this carbon originates from within the ecosystem itself (autochthonous carbon) while some originates from outside the ecosystem (allochthonous carbon). The exact proportion of autochthonous versus allochthonous carbon is difficult to determine, and differs by site, but is important when trying to account for all the carbon fluxes in a system. What’s more, carbon can move laterally and vertically in, out, and through these systems, creating additional complexity for carbon accounting.
Of these three ecosystems, in terms of current global extent, current carbon storage, and carbon sequestration potential, mangrove forests are the best understood followed by salt marshes. Seagrass beds are the least well understood both in spatial extent and ultimate carbon sequestration potential.
Restoration and its limitations: CDR pathways within coastal vegetated ecosystems are primarily concerned with restoration – the act of bringing an ecosystem back as nearly as possible to its original condition. However, the goal of restoration may be unattainable for several reasons, including irreversible land and hydrological (starved of freshwater input) change, conditions that do not permit rehabilitation, and the inherent spatial limitations for ecosystems that occupy specific niches. Another challenge is the host of known and unknown consequences of climate change which may impact the feasibility and efficacy of restoration efforts. Monitoring of restoration projects will also be critical both as important data points for studies and predictive science as well as for any involvement with carbon markets. There has been historical reluctance from both government agencies and private developers to fund long-term monitoring, however this will be crucial for informed decision making about where and how to implement restoration projects.
Net sequestration of CO2e and its storage durability are key metrics when considering restoration projects for CDR. Ideally, monitoring of restoration projects should feed back into adaptive management practices, where management techniques are adjusted though an iterative process in response to other variables such as climate change.
Mangrove forests, salt marshes, and seagrass beds grow where land meets the sea and store carbon in their vegetation and underlying sediments/soils. The sediments/soils can store both organic and inorganic carbon. Some of this carbon originates from within the ecosystem itself (autochthonous carbon) while some originates from outside the ecosystem (allochthonous carbon). The exact proportion of autochthonous versus allochthonous carbon is difficult to determine, and differs by site, but is important when trying to account for all the carbon fluxes in a system. What’s more, carbon can move laterally and vertically in, out, and through these systems, creating additional complexity for carbon accounting.
Of these three ecosystems, in terms of current global extent, current carbon storage, and carbon sequestration potential, mangrove forests are the best understood followed by salt marshes. Seagrass beds are the least well understood both in spatial extent and ultimate carbon sequestration potential.
Restoration and its limitations: CDR pathways within coastal vegetated ecosystems are primarily concerned with restoration – the act of bringing an ecosystem back as nearly as possible to its original condition[1]As defined by the US National Research Council (1992) . However, the goal of restoration may be unattainable for several reasons, including irreversible land and hydrological (starved of freshwater input) change, conditions that do not permit rehabilitation, and the inherent spatial limitations for ecosystems that occupy specific niches[2]Adam, Paul. “Salt Marsh Restoration.” Coastal Wetlands, 2019, pp. 817–861, https://doi.org/10.1016/b978-0-444-63893-9.00023-x. . Another challenge is the host of known and unknown consequences of climate change which may impact the feasibility and efficacy of restoration efforts. Monitoring of restoration projects will also be critical both as important data points for studies and predictive science as well as for any involvement with carbon markets. There has been historical reluctance from both government agencies and private developers to fund long-term monitoring[3]Adam, Paul. “Salt Marsh Restoration.” Coastal Wetlands, 2019, pp. 817–861, https://doi.org/10.1016/b978-0-444-63893-9.00023-x. , however this will be crucial for informed decision making about where and how to implement restoration projects.
Net sequestration of CO2e and its storage durability are key metrics when considering restoration projects for CDR. Ideally, monitoring of restoration projects should feed back into adaptive management practices, where management techniques are adjusted though an iterative process in response to other variables such as climate change.
Mangrove Forests
Mechanism for CDR
- Restoring hydrology: Restoration of hydrology, through reversing drainage or removing tidal restrictions may assist in the recovery and expansion of existing mangrove sites. This path may be met with more resistance (especially political and social) than other types of restoration efforts.
- Planting mangrove seedlings: This is one of the most common restoration techniques, however, large-scale planting efforts have often failed (Zimmer et al., 2022) (many of these shortcomings a result of projects that do not adequately consider environmental and socioeconomic conditions (Lovelock et al., 2022)) and smaller projects may not deliver carbon storage on climate-relevant scales (Lovelock et al., 2022).
-
- Research is being done on the efficacy of automated mangrove planting via drones (AlRaisi et al., 2023; Ayub et al., 2023). This work is still in early stages but may provide lower cost options for planting efforts, especially in difficult to access locations.
- Restoration efforts should be de-risked in smaller projects before moving to larger projects. This will require investment in capacity-building in communities and institutions, and mechanisms to match restoration opportunities with prospective supporters and investors (Lovelock et al., 2022).
CDR Potential
Estimated Sequestration Potential: 0.028 – 0.172 Gt CO2e/year (Hoegh-Guldberg et al., 2023)
Sequestration Durability: Decades — 1,000 years (Cross et al., 2023) (Durability of sequestered carbon will depend on multiple variables and can vary greatly between individual ecosystems)
Challenges
- Accounting:
-
- Estimating future sequestration potentials or future rates of carbon sequestration is more difficult than estimating their current global extent and storage.
- While there is general scientific consensus that mangroves are net sinks of CO2 (Rosentreter et al., 2023), there is growing research that indicates production of CH4 and N2O nonuniformly across habitats. In order to have accurate calculations of greenhouse gas budgets, these emissions will need to be included in the accounting (Collins et al., 2022).
- Current estimates of sequestration potential do not account for the effects of climate change on the ecosystem (e.g., warming, sea level rise, coastal squeeze, increased tropical storms).
- Mangroves are inextricably connected to other marine ecosystems like seagrasses and coral reefs, complicating the definition of boundaries for estimating “additional” carbon sequestration and introducing other complications, such as figuring out how much carbon is autochthonous and how much is allochthonous. Additionally, many carbon markets currently require that all carbon be autochthonous to count toward credits or have poorly defined frameworks for whether to credit for allochthonous carbon that accumulates on site as a result of restoration or management actions.
- Restoration:
-
- There are high costs associated with restoration efforts (Vanderklift et al., 2018).
- Mangrove ecosystems are heterogeneous across geographies, and this must be accounted for when assessing individual habitat potential for carbon sequestration. (e.g., findings from one location/ecosystem may not be directly applicable to other locations/ecosystems
- There is work being done to use unmanned aerial systems (UAS) to assess mangrove estuaries on the Pacific Coast of Costa Rica (Yaney-Keller et al., 2019) and in Malaysia (Otero et al., 2018), enabling monitoring of difficult-to-access locations. This type of innovation may be important for monitoring and verification of restored mangrove forests.
Key Knowledge Gaps
- Quantitative understanding of the impacts of climate change on mangrove ecosystems and how these may affect restoration efforts.
- Incomplete understanding of greenhouse gas production and release (e.g., CH4 and N2O) by and from mangroves and how this impacts net carbon removal.
- Uncertainty around the durability of carbon stored in mangroves and their sediments as well as carbon burial rates (Williamson & Gattuso, 2022).
First-Order Priorities
*Adapted from the Environmental Defense Fund report on Coastal Natural Climate Solutions (Collins et al., 2022)
- Improve predictions of the effects of climate change on mangrove forests*
- Predictions needed include:
- Effects of increased tropical storm intensity and frequency on the destruction and degradation of mangrove ecosystems
- The timescales over which any previously sequestered carbon could be released to the atmosphere
- Understand/map the potential spatial extent of mangrove forests given climate change impacts on existing habitats and poleward migration of mangroves with future warming.
- Predictions needed include:
- Improved, spatially explicit modeling approaches to predict greenhouse gas fluxes and net carbon sequestration*
- Models should function across different mangrove ecosystems whose sediment dynamics cannot be resolved solely using imagery taken from space or aircraft.
- The development of carbon crediting projects requires we know not only how much carbon a mangrove ecosystem contains in its above and belowground biomass, but also the magnitude of various greenhouse gas fluxes from underlying soils or sediments at present and in the future.
- These models should account for future ecosystem state due to the interactive effects of climate change and predicted land-use changes, including the phenomenon of coastal squeeze, the prevention of expansion due to rural areas on one side and the ocean on the other.
- Create studies designed to better differentiate between carbon originating from within the system (autochthonous) and carbon originating from elsewhere (allochthonous)*
- The ability to distinguish among carbon sources is particularly critical since methodologies developed under the voluntary carbon market standards require the deduction of allochthonous carbon from any claimed credit.
- Accelerate the development of technology and biotechnology that can help scale restoration efforts
- Optimize drones and autonomous robots for increased scalability of mangrove planting.
- Test biological engineering techniques to enhance carbon storage within mangrove forests.
Mechanism for CDR
- Restoring hydrology: Restoration of hydrology, through reversing drainage or removing tidal restrictions may assist in the recovery and expansion of existing mangrove sites. This path may be met with more resistance (especially political and social) than other types of restoration efforts.
- Planting mangrove seedlings: This is one of the most common restoration techniques, however, large-scale planting efforts have often failed (Zimmer et al., 2022) (many of these shortcomings a result of projects that do not adequately consider environmental and socioeconomic conditions (Lovelock et al., 2022)) and smaller projects may not deliver carbon storage on climate-relevant scales (Lovelock et al., 2022).
-
- Research is being done on the efficacy of automated mangrove planting via drones (AlRaisi et al., 2023; Ayub et al., 2023). This work is still in early stages but may provide lower cost options for planting efforts, especially in difficult to access locations.
- Restoration efforts should be de-risked in smaller projects before moving to larger projects. This will require investment in capacity-building in communities and institutions, and mechanisms to match restoration opportunities with prospective supporters and investors (Lovelock et al., 2022).
CDR Potential
Estimated Sequestration Potential: 0.028 – 0.172 Gt CO2e/year (Hoegh-Guldberg et al., 2023)
Sequestration Durability: Decades -- 1,000 years (Cross et al., 2023) (Durability of sequestered carbon will depend on multiple variables and can vary greatly between individual ecosystems)
Challenges
- Accounting:
-
- Estimating future sequestration potentials or future rates of carbon sequestration is more difficult than estimating their current global extent and storage.
- While there is general scientific consensus that mangroves are net sinks of CO2 (Rosentreter et al., 2023), there is growing research that indicates production of CH4 and N2O nonuniformly across habitats. In order to have accurate calculations of greenhouse gas budgets, these emissions will need to be included in the accounting (Collins et al., 2022).
- Current estimates of sequestration potential do not account for the effects of climate change on the ecosystem (e.g., warming, sea level rise, coastal squeeze, increased tropical storms).
- Mangroves are inextricably connected to other marine ecosystems like seagrasses and coral reefs, complicating the definition of boundaries for estimating “additional” carbon sequestration and introducing other complications, such as figuring out how much carbon is autochthonous and how much is allochthonous. Additionally, many carbon markets currently require that all carbon be autochthonous to count toward credits or have poorly defined frameworks for whether to credit for allochthonous carbon that accumulates on site as a result of restoration or management actions.
- Restoration:
-
- There are high costs associated with restoration efforts (Vanderklift et al., 2018).
- Mangrove ecosystems are heterogeneous across geographies, and this must be accounted for when assessing individual habitat potential for carbon sequestration. (e.g., findings from one location/ecosystem may not be directly applicable to other locations/ecosystems
- There is work being done to use unmanned aerial systems (UAS) to assess mangrove estuaries on the Pacific Coast of Costa Rica (Yaney-Keller et al., 2019) and in Malaysia (Otero et al., 2018), enabling monitoring of difficult-to-access locations. This type of innovation may be important for monitoring and verification of restored mangrove forests.
Key Knowledge Gaps
- Quantitative understanding of the impacts of climate change on mangrove ecosystems and how these may affect restoration efforts.
- Incomplete understanding of greenhouse gas production and release (e.g., CH4 and N2O) by and from mangroves and how this impacts net carbon removal.
- Uncertainty around the durability of carbon stored in mangroves and their sediments as well as carbon burial rates (Williamson & Gattuso, 2022).
First-Order Priorities
*Adapted from the Environmental Defense Fund report on Coastal Natural Climate Solutions (Collins et al., 2022)
- Improve predictions of the effects of climate change on mangrove forests*
- Predictions needed include:
- Effects of increased tropical storm intensity and frequency on the destruction and degradation of mangrove ecosystems
- The timescales over which any previously sequestered carbon could be released to the atmosphere
- Understand/map the potential spatial extent of mangrove forests given climate change impacts on existing habitats and poleward migration of mangroves with future warming.
- Predictions needed include:
- Improved, spatially explicit modeling approaches to predict greenhouse gas fluxes and net carbon sequestration*
- Models should function across different mangrove ecosystems whose sediment dynamics cannot be resolved solely using imagery taken from space or aircraft.
- The development of carbon crediting projects requires we know not only how much carbon a mangrove ecosystem contains in its above and belowground biomass, but also the magnitude of various greenhouse gas fluxes from underlying soils or sediments at present and in the future.
- These models should account for future ecosystem state due to the interactive effects of climate change and predicted land-use changes, including the phenomenon of coastal squeeze, the prevention of expansion due to rural areas on one side and the ocean on the other.
- Create studies designed to better differentiate between carbon originating from within the system (autochthonous) and carbon originating from elsewhere (allochthonous)*
- The ability to distinguish among carbon sources is particularly critical since methodologies developed under the voluntary carbon market standards require the deduction of allochthonous carbon from any claimed credit.
- Accelerate the development of technology and biotechnology that can help scale restoration efforts
- Optimize drones and autonomous robots for increased scalability of mangrove planting.
- Test biological engineering techniques to enhance carbon storage within mangrove forests.
Mechanism for CDR
- Restoring hydrology: Restoration of hydrology, through reversing drainage or removing tidal restrictions may assist in the recovery and expansion of existing mangrove sites. This path may be met with more resistance (especially political and social) than other types of restoration efforts.
- Planting mangrove seedlings: This is one of the most common restoration techniques, however, large-scale planting efforts have often failed, (many of these shortcomings a result of projects that do not adequately consider environmental and socioeconomic conditions) and smaller projects may not deliver carbon storage on climate-relevant scales.
-
- Research is being done on the efficacy of automated mangrove planting via drones,. This work is still in early stages but may provide lower cost options for planting efforts, especially in difficult to access locations.
- Restoration efforts should be de-risked in smaller projects before moving to larger projects. This will require investment in capacity-building in communities and institutions, and mechanisms to match restoration opportunities with prospective supporters and investors.
CDR Potential
Estimated Sequestration Potential: 0.028 – 0.172 Gt CO2e/year
Sequestration Durability: Decades -- 1,000 years (Durability of sequestered carbon will depend on multiple variables and can vary greatly between individual ecosystems)
Challenges
- Accounting:
-
- Estimating future sequestration potentials or future rates of carbon sequestration is more difficult than estimating their current global extent and storage.
- While there is general scientific consensus that mangroves are net sinks of CO2, there is growing research that indicates production of CH4 and N2O nonuniformly across habitats. In order to have accurate calculations of greenhouse gas budgets, these emissions will need to be included in the accounting.
- Current estimates of sequestration potential do not account for the effects of climate change on the ecosystem (e.g., warming, sea level rise, coastal squeeze, increased tropical storms).
- Mangroves are inextricably connected to other marine ecosystems like seagrasses and coral reefs, complicating the definition of boundaries for estimating “additional” carbon sequestration and introducing other complications, such as figuring out how much carbon is autochthonous and how much is allochthonous. Additionally, many carbon markets currently require that all carbon be autochthonous to count toward credits or have poorly defined frameworks for whether to credit for allochthonous carbon that accumulates on site as a result of restoration or management actions.
- Restoration:
-
- There are high costs associated with restoration efforts.
- Mangrove ecosystems are heterogeneous across geographies, and this must be accounted for when assessing individual habitat potential for carbon sequestration. (e.g., findings from one location/ecosystem may not be directly applicable to other location/ecosystems
- There is work being done to use unmanned aerial systems (UAS) to assess mangrove estuaries on the Pacific Coast of Costa Rica and in Malaysia, enabling monitoring of difficult to access locations. This type of innovation may be important for monitoring and verification of restored mangrove forests.
Key Knowledge Gaps
- Quantitative understanding of the impacts of climate change on mangrove ecosystems and how these may affect restoration efforts.
- Incomplete understanding of greenhouse gas production and release (e.g., CH4 and N2O) by and from mangroves and how this impacts net carbon removal.
- Uncertainty around durability of carbon stored in mangroves and their sediments as well as carbon burial rates.
First-Order Priorities
*Adapted from the Environmental Defense Fund report on Coastal Natural Climate Solutions
- Improve predictions of the effects of climate change on mangrove forests*
- Predictions needed include:
- Effects of increased tropical storm intensity and frequency on the destruction and degradation of mangrove ecosystems
- The timescales over which any previously sequestered carbon could be released to the atmosphere
- Understand/map the potential spatial extent of mangrove forests given climate change impacts on existing habitats and poleward migration of mangroves with future warming.
- Predictions needed include:
- Improved, spatially explicit modeling approaches to predict greenhouse gas fluxes and net carbon sequestration*
- Models should function across different mangrove ecosystems whose sediment dynamics cannot be resolved solely using imagery taken from space or aircraft.
- The development of carbon crediting projects requires we know not only how much carbon a mangrove ecosystem contains in its above and belowground biomass, but also the magnitude of various greenhouse gas fluxes from underlying soils or sediments at present and in the future.
- These models should account for future ecosystem state due to the interactive effects of climate change and predicted land-use changes, including the phenomenon of coastal squeeze, the prevention of expansion due to rural areas on one side and the ocean on the other.
- Create studies designed to better differentiate between carbon originating from within the system (autochthonous) and carbon originating from elsewhere (allochthonous)*
- The ability to distinguish among carbon sources is particularly critical since methodologies developed under the voluntary carbon market standards require deduction of allochthonous carbon from any claimed credit.
- Accelerate the development of technology and biotechnology that can help scale restoration efforts
- Optimize drones and autonomous robots for increased scalability of mangrove planting.
- Test biological engineering techniques to enhance carbon storage within mangrove forests.
Mechanism for CDR
- Restoring hydrology: Restoration of hydrology, through reversing drainage or removing tidal restrictions may assist in the recovery and expansion of existing mangrove sites. This path may be met with more resistance (especially political and social) than other types of restoration efforts.
- Planting mangrove seedlings: This is one of the most common restoration techniques, however, large-scale planting efforts have often failed[1]Zimmer M, Ajonina GN, Amir AA, Cragg SM, Crooks S, Dahdouh-Guebas F, Duke NC, Fratini S, Friess DA, Helfer V, Huxham M, Kathiresan K, Kodikara KAS, Koedam N, Lee SY, Mangora MM, Primavera J, Satyanarayana B, Yong JWH and Wodehouse D (2022) When nature needs a helping hand: Different levels of human intervention for mangrove (re-)establishment. Front. For. Glob. Change 5:784322. doi: 10.3389/ffgc.2022.784322 , (many of these shortcomings a result of projects that do not adequately consider environmental and socioeconomic conditions[2]Lovelock CE,Barbier E,Duarte CM(2022) Tackling the mangrove restoration challenge. PLoS Biol20(10): e3001836. https://doi.org/10.1371/journal.pbio.3001836 ) and smaller projects may not deliver carbon storage on climate-relevant scales[3]Lovelock CE, Barbier E, Duarte CM (2022) Tackling the mangrove restoration challenge. PLoS Biol 20(10): e3001836. https://doi.org/10.1371/journal.pbio.3001836 .
-
- Research is being done on the efficacy of automated mangrove planting via drones[4]AlRaisi, A. A., et al. “Restoration technology hand in hand with nature-based solutions: ADNOC’s drone led Mangrove Restoration Project.” Day 1 Mon, October 02, 2023, 2023, https://doi.org/10.2118/215963-ms. ,[5]Ayub, Ayub Sugara, et al. “Mapping the potential of mangrove planting in the rehabilitation of coastal ecosystems using drone technology.” Journal of Sylva Indonesiana, vol. 6, no. 02, 2023, pp. 164–177, https://doi.org/10.32734/jsi.v6i02.10515. . This work is still in early stages but may provide lower cost options for planting efforts, especially in difficult to access locations.
- Restoration efforts should be de-risked in smaller projects before moving to larger projects. This will require investment in capacity-building in communities and institutions, and mechanisms to match restoration opportunities with prospective supporters and investors[6]Lovelock CE, Barbier E, Duarte CM (2022) Tackling the mangrove restoration challenge. PLoS Biol 20(10): e3001836. https://doi.org/10.1371/journal.pbio.3001836 .
CDR Potential
Estimated Sequestration Potential: 0.028 – 0.172 Gt CO2e/year[7]Hoegh-Guldberg, O., Northrop, E. et al. 2023. "The ocean as a solution to climate change: Updated opportunities for action." Special Report. Washington, DC: World Resources Institute. Available online at https://oceanpanel.org/publication/ocean-solutions-to-climate-change
Sequestration Durability: Decades -- 1,000 years[8]Cross, J.N., Sweeney, C., Jewett, E.B., Feely, R.A., McElhany, P., Carter, B., Stein, T., Kitch, G.D., and Gledhill, D.K., 2023. Strategy for NOAA Carbon Dioxide Removal Research: A white paper documenting a potential NOAA CDR Science Strategy as an element of NOAA’s Climate Interventions Portfolio. NOAA Special Report. NOAA, Washington DC. DOI: 10.25923/gzke-8730 (Durability of sequestered carbon will depend on multiple variables and can vary greatly between individual ecosystems)
Challenges
- Accounting:
-
- Estimating future sequestration potentials or future rates of carbon sequestration is more difficult than estimating their current global extent and storage.
- While there is general scientific consensus that mangroves are net sinks of CO2[9]Rosentreter, Judith A., et al. “Coastal vegetation and estuaries are collectively a greenhouse gas sink.” Nature Climate Change, vol. 13, no. 6, 2023, pp. 579–587, https://doi.org/10.1038/s41558-023-01682-9. , there is growing research that indicates production of CH4 and N2O nonuniformly across habitats. In order to have accurate calculations of greenhouse gas budgets, these emissions will need to be included in the accounting[10]Collins, J. R., Boenish, R. E., Kleisner, K. M., Rader, D. N., Fujita, R. M., and Moritsch M. M. 2022. Coastal natural climate solutions: an assessment of scientific knowledge surrounding pathways for carbon dioxide removal and avoided emissions in nearshore blue carbon ecosystems. Environmental Defense Fund, New York, NY. https://www.edf.org/sites/default/files/2022-10/Coastal%20Natural%20Climate%20Solutions.pdf .
- Current estimates of sequestration potential do not account for the effects of climate change on the ecosystem (e.g., warming, sea level rise, coastal squeeze, increased tropical storms).
- Mangroves are inextricably connected to other marine ecosystems like seagrasses and coral reefs, complicating the definition of boundaries for estimating “additional” carbon sequestration and introducing other complications, such as figuring out how much carbon is autochthonous and how much is allochthonous. Additionally, many carbon markets currently require that all carbon be autochthonous to count toward credits or have poorly defined frameworks for whether to credit for allochthonous carbon that accumulates on site as a result of restoration or management actions.
- Restoration:
-
- There are high costs associated with restoration efforts[11]Vanderklift M, Steven A, Marcos-Martinez R, Gorman D (2018) Achieving carbon offsets through blue carbon: a review of needs and opportunities relevant to the Australian seafood industry, FRDC Final Report 2018/060. CSIRO Oceans and Atmosphere, Crawley, November 2018. .
- Mangrove ecosystems are heterogeneous across geographies, and this must be accounted for when assessing individual habitat potential for carbon sequestration. (e.g., findings from one location/ecosystem may not be directly applicable to other location/ecosystems
- There is work being done to use unmanned aerial systems (UAS) to assess mangrove estuaries on the Pacific Coast of Costa Rica[12]Yaney-Keller A, Santidrián Tomillo P, Marshall JM, Paladino FV (2019) Using Unmanned Aerial Systems (UAS) to assay mangrove estuaries on the Pacific coast of Costa Rica. PLoS ONE 14(6): e0217310. https://doi.org/10.1371/journal.pone.0217310 and in Malaysia[13]Otero, Viviana, et al. “Managing mangrove forests from the Sky: Forest Inventory using field data and Unmanned Aerial Vehicle (UAV) imagery in the Matang Mangrove Forest Reserve, peninsular Malaysia.” Forest Ecology and Management, vol. 411, 2018, pp. 35–45, https://doi.org/10.1016/j.foreco.2017.12.049. , enabling monitoring of difficult to access locations. This type of innovation may be important for monitoring and verification of restored mangrove forests.
Key Knowledge Gaps
- Quantitative understanding of the impacts of climate change on mangrove ecosystems and how these may affect restoration efforts.
- Incomplete understanding of greenhouse gas production and release (e.g., CH4 and N2O) by and from mangroves and how this impacts net carbon removal.
- Uncertainty around durability of carbon stored in mangroves and their sediments as well as carbon burial rates[14]Williamson P and Gattuso J-P (2022) Carbon Removal Using Coastal Blue Carbon Ecosystems Is Uncertain and Unreliable, With Questionable Climatic Cost-Effectiveness. Front. Clim. 4:853666. doi: 10.3389/fclim.2022.853666 .
First-Order Priorities
*Adapted from the Environmental Defense Fund report on Coastal Natural Climate Solutions[15]Collins, J. R., Boenish, R. E., Kleisner, K. M., Rader, D. N., Fujita, R. M., and Moritsch M. M. 2022. Coastal natural climate solutions: an assessment of scientific knowledge surrounding pathways for carbon dioxide removal and avoided emissions in nearshore blue carbon ecosystems. Environmental Defense Fund, New York, NY. https://www.edf.org/sites/default/files/2022-10/Coastal%20Natural%20Climate%20Solutions.pdf
- Improve predictions of the effects of climate change on mangrove forests*
- Predictions needed include:
- Effects of increased tropical storm intensity and frequency on the destruction and degradation of mangrove ecosystems
- The timescales over which any previously sequestered carbon could be released to the atmosphere
- Understand/map the potential spatial extent of mangrove forests given climate change impacts on existing habitats and poleward migration of mangroves with future warming.
- Predictions needed include:
- Improved, spatially explicit modeling approaches to predict greenhouse gas fluxes and net carbon sequestration*
- Models should function across different mangrove ecosystems whose sediment dynamics cannot be resolved solely using imagery taken from space or aircraft.
- The development of carbon crediting projects requires we know not only how much carbon a mangrove ecosystem contains in its above and belowground biomass, but also the magnitude of various greenhouse gas fluxes from underlying soils or sediments at present and in the future.
- These models should account for future ecosystem state due to the interactive effects of climate change and predicted land-use changes, including the phenomenon of coastal squeeze, the prevention of expansion due to rural areas on one side and the ocean on the other.
- Create studies designed to better differentiate between carbon originating from within the system (autochthonous) and carbon originating from elsewhere (allochthonous)*
- The ability to distinguish among carbon sources is particularly critical since methodologies developed under the voluntary carbon market standards require deduction of allochthonous carbon from any claimed credit.
- Accelerate the development of technology and biotechnology that can help scale restoration efforts
- Optimize drones and autonomous robots for increased scalability of mangrove planting.
- Test biological engineering techniques to enhance carbon storage within mangrove forests.
Mechanism for CDR
- Restoring hydrology: Restoration of hydrology, through reversing drainage or removing tidal restrictions may assist in the recovery and expansion of existing mangrove sites. This path may be met with more resistance (especially political and social) than other types of restoration efforts.
- Planting mangrove seedlings: This is one of the most common restoration techniques, however, large-scale planting efforts have often failed[1]Zimmer M, Ajonina GN, Amir AA, Cragg SM, Crooks S, Dahdouh-Guebas F, Duke NC, Fratini S, Friess DA, Helfer V, Huxham M, Kathiresan K, Kodikara KAS, Koedam N, Lee SY, Mangora MM, Primavera J, Satyanarayana B, Yong JWH and Wodehouse D (2022) When nature needs a helping hand: Different levels of human intervention for mangrove (re-)establishment. Front. For. Glob. Change 5:784322. doi: 10.3389/ffgc.2022.784322 , (many of these shortcomings a result of projects that do not adequately consider environmental and socioeconomic conditions[2]Lovelock CE,Barbier E,Duarte CM(2022) Tackling the mangrove restoration challenge. PLoS Biol20(10): e3001836. https://doi.org/10.1371/ journal.pbio.3001836 ) and smaller projects may not deliver carbon storage on climate-relevant scales[3]Lovelock CE, Barbier E, Duarte CM (2022) Tackling the mangrove restoration challenge. PLoS Biol 20(10): e3001836. https://doi.org/10.1371/ journal.pbio.3001836 .
-
- Research is being done on the efficacy of automated mangrove planting via drones[4]AlRaisi, A. A., et al. “Restoration technology hand in hand with nature-based solutions: ADNOC’s drone led Mangrove Restoration Project.” Day 1 Mon, October 02, 2023, 2023, https://doi.org/10.2118/215963-ms. ,[5]Ayub, Ayub Sugara, et al. “Mapping the potential of mangrove planting in the rehabilitation of coastal ecosystems using drone technology.” Journal of Sylva Indonesiana, vol. 6, no. 02, 2023, pp. 164–177, https://doi.org/10.32734/jsi.v6i02.10515. . This work is still in early stages but may provide lower cost options for planting efforts, especially in difficult to access locations.
- Restoration efforts should be de-risked in smaller projects before moving to larger projects. This will require investment in capacity-building in communities and institutions, and mechanisms to match restoration opportunities with prospective supporters and investors[6]Lovelock CE, Barbier E, Duarte CM (2022) Tackling the mangrove restoration challenge. PLoS Biol 20(10): e3001836. https://doi.org/10.1371/ journal.pbio.3001836 .
CDR Potential
Estimated Sequestration Potential: 0.028 – 0.172 Gt CO2e/year[7]Hoegh-Guldberg, O., Northrop, E. et al. 2023. "The ocean as a solution to climate change: Updated opportunities for action." Special Report. Washington, DC: World Resources Institute. Available online at https://oceanpanel.org/publication/ocean-solutions-to-climate-change
Sequestration Durability: Decades -- 1,000 years[8]Cross, J.N., Sweeney, C., Jewett, E.B., Feely, R.A., McElhany, P., Carter, B., Stein, T., Kitch, G.D., and Gledhill, D.K., 2023. Strategy for NOAA Carbon Dioxide Removal Research: A white paper documenting a potential NOAA CDR Science Strategy as an element of NOAA’s Climate Interventions Portfolio. NOAA Special Report. NOAA, Washington DC. DOI: 10.25923/gzke-8730 (Durability of sequestered carbon will depend on multiple variables and can vary greatly between individual ecosystems)
Challenges
- Accounting:
-
- Estimating future sequestration potentials or future rates of carbon sequestration is more difficult than estimating their current global extent and storage.
- While there is general scientific consensus that mangroves are net sinks of CO2[9]Rosentreter, Judith A., et al. “Coastal vegetation and estuaries are collectively a greenhouse gas sink.” Nature Climate Change, vol. 13, no. 6, 2023, pp. 579–587, https://doi.org/10.1038/s41558-023-01682-9. , there is growing research that indicates production of CH4 and N2O nonuniformly across habitats. In order to have accurate calculations of greenhouse gas budgets, these emissions will need to be included in the accounting[10]Collins, J. R., Boenish, R. E., Kleisner, K. M., Rader, D. N., Fujita, R. M., and Moritsch M. M. 2022. Coastal natural climate solutions: an assessment of scientific knowledge surrounding pathways for carbon dioxide removal and avoided emissions in nearshore blue carbon ecosystems. Environmental Defense Fund, New York, NY. https://www.edf.org/sites/default/files/2022-10/Coastal%20Natural%20Climate%20Solutions.pdf .
- Current estimates of sequestration potential do not account for the effects of climate change on the ecosystem (e.g., warming, sea level rise, coastal squeeze, increased tropical storms).
- Mangroves are inextricably connected to other marine ecosystems like seagrasses and coral reefs, complicating the definition of boundaries for estimating “additional” carbon sequestration and introducing other complications, such as figuring out how much carbon is autochthonous and how much is allochthonous. Additionally, many carbon markets currently require that all carbon be autochthonous to count toward credits or have poorly defined frameworks for whether to credit for allochthonous carbon that accumulates on site as a result of restoration or management actions.
- Restoration:
-
- There are high costs associated with restoration efforts[11]Vanderklift M, Steven A, Marcos-Martinez R, Gorman D (2018) Achieving carbon offsets through blue carbon: a review of needs and opportunities relevant to the Australian seafood industry, FRDC Final Report 2018/060. CSIRO Oceans and Atmosphere, Crawley, November 2018. .
- Mangrove ecosystems are heterogeneous across geographies, and this must be accounted for when assessing individual habitat potential for carbon sequestration. (e.g., findings from one location/ecosystem may not be directly applicable to other location/ecosystems
- There is work being done to use unmanned aerial systems (UAS) to assess mangrove estuaries on the Pacific Coast of Costa Rica[12]Yaney-Keller A, Santidrián Tomillo P, Marshall JM, Paladino FV (2019) Using Unmanned Aerial Systems (UAS) to assay mangrove estuaries on the Pacific coast of Costa Rica. PLoS ONE 14(6): e0217310. https://doi.org/10.1371/journal.pone.0217310 and in Malaysia[13]Otero, Viviana, et al. “Managing mangrove forests from the Sky: Forest Inventory using field data and Unmanned Aerial Vehicle (UAV) imagery in the Matang Mangrove Forest Reserve, peninsular Malaysia.” Forest Ecology and Management, vol. 411, 2018, pp. 35–45, https://doi.org/10.1016/j.foreco.2017.12.049. , enabling monitoring of difficult to access locations. This type of innovation may be important for monitoring and verification of restored mangrove forests.
Key Knowledge Gaps
- Quantitative understanding of the impacts of climate change on mangrove ecosystems and how these may affect restoration efforts.
- Incomplete understanding of greenhouse gas production and release (e.g., CH4 and N2O) by and from mangroves and how this impacts net carbon removal.
- Uncertainty around durability of carbon stored in mangroves and their sediments as well as carbon burial rates[14]Williamson P and Gattuso J-P (2022) Carbon Removal Using Coastal Blue Carbon Ecosystems Is Uncertain and Unreliable, With Questionable Climatic Cost-Effectiveness. Front. Clim. 4:853666. doi: 10.3389/fclim.2022.853666 .
First-Order Priorities
*Adapted from the Environmental Defense Fund report on Coastal Natural Climate Solutions[15]Collins, J. R., Boenish, R. E., Kleisner, K. M., Rader, D. N., Fujita, R. M., and Moritsch M. M. 2022. Coastal natural climate solutions: an assessment of scientific knowledge surrounding pathways for carbon dioxide removal and avoided emissions in nearshore blue carbon ecosystems. Environmental Defense Fund, New York, NY. https://www.edf.org/sites/default/files/2022-10/Coastal%20Natural%20Climate%20Solutions.pdf
- Improve predictions of the effects of climate change on mangrove forests*
- Predictions needed include:
- Effects of increased tropical storm intensity and frequency on the destruction and degradation of mangrove ecosystems
- The timescales over which any previously sequestered carbon could be released to the atmosphere
- Understand/map the potential spatial extent of mangrove forests given climate change impacts on existing habitats and poleward migration of mangroves with future warming.
- Predictions needed include:
- Improved, spatially explicit modeling approaches to predict greenhouse gas fluxes and net carbon sequestration*
- Models should function across different mangrove ecosystems whose sediment dynamics cannot be resolved solely using imagery taken from space or aircraft.
- The development of carbon crediting projects requires we know not only how much carbon a mangrove ecosystem contains in its above and belowground biomass, but also the magnitude of various greenhouse gas fluxes from underlying soils or sediments at present and in the future.
- These models should account for future ecosystem state due to the interactive effects of climate change and predicted land-use changes, including the phenomenon of coastal squeeze, the prevention of expansion due to rural areas on one side and the ocean on the other.
- Create studies designed to better differentiate between carbon originating from within the system (autochthonous) and carbon originating from elsewhere (allochthonous)*
- The ability to distinguish among carbon sources is particularly critical since methodologies developed under the voluntary carbon market standards require deduction of allochthonous carbon from any claimed credit.
- Accelerate the development of technology and biotechnology that can help scale restoration efforts
- Optimize drones and autonomous robots for increased scalability of mangrove planting.
- Test biological engineering techniques to enhance carbon storage within mangrove forests.
Mechanism for CDR
- Restoring hydrology: Restoration of hydrology, through reversing drainage or removing tidal restrictions may assist in the recovery and expansion of existing mangrove sites. This path may be met with more resistance (especially political and social) than other types of restoration efforts.
- Planting mangrove seedlings: This is one of the most common restoration techniques, however, large-scale planting efforts have often failed[1]Zimmer M, Ajonina GN, Amir AA, Cragg SM, Crooks S, Dahdouh-Guebas F, Duke NC, Fratini S, Friess DA, Helfer V, Huxham M, Kathiresan K, Kodikara KAS, Koedam N, Lee SY, Mangora MM, Primavera J, Satyanarayana B, Yong JWH and Wodehouse D (2022) When nature needs a helping hand: Different levels of human intervention for mangrove (re-)establishment. Front. For. Glob. Change 5:784322. doi: 10.3389/ffgc.2022.784322 , (many of these shortcomings a result of projects that do not adequately consider environmental and socioeconomic conditions[2]Lovelock CE,Barbier E,Duarte CM(2022) Tackling the mangrove restoration challenge. PLoS Biol20(10): e3001836. https://doi.org/10.1371/ journal.pbio.3001836 ) and smaller projects may not deliver carbon storage on climate-relevant scales[3]Lovelock CE, Barbier E, Duarte CM (2022) Tackling the mangrove restoration challenge. PLoS Biol 20(10): e3001836. https://doi.org/10.1371/ journal.pbio.3001836 .
-
- Research is being done on the efficacy of automated mangrove planting via drones[4]AlRaisi, A. A., et al. “Restoration technology hand in hand with nature-based solutions: ADNOC’s drone led Mangrove Restoration Project.” Day 1 Mon, October 02, 2023, 2023, https://doi.org/10.2118/215963-ms. ,[5]Ayub, Ayub Sugara, et al. “Mapping the potential of mangrove planting in the rehabilitation of coastal ecosystems using drone technology.” Journal of Sylva Indonesiana, vol. 6, no. 02, 2023, pp. 164–177, https://doi.org/10.32734/jsi.v6i02.10515. . This work is still in early stages but may provide lower cost options for planting efforts, especially in difficult to access locations.
- Restoration efforts should be de-risked in smaller projects before moving to larger projects. This will require investment in capacity-building in communities and institutions, and mechanisms to match restoration opportunities with prospective supporters and investors[6]Lovelock CE, Barbier E, Duarte CM (2022) Tackling the mangrove restoration challenge. PLoS Biol 20(10): e3001836. https://doi.org/10.1371/ journal.pbio.3001836 .
CDR Potential
Estimated Sequestration Potential: 0.028 – 0.172 Gt CO2e/year[7]Hoegh-Guldberg, O., Northrop, E. et al. 2023. "The ocean as a solution to climate change: Updated opportunities for action." Special Report. Washington, DC: World Resources Institute. Available online at https://oceanpanel.org/publication/ocean-solutions-to-climate-change
Sequestration Durability: Decades -- 1,000 years[8]Cross, J.N., Sweeney, C., Jewett, E.B., Feely, R.A., McElhany, P., Carter, B., Stein, T., Kitch, G.D., and Gledhill, D.K., 2023. Strategy for NOAA Carbon Dioxide Removal Research: A white paper documenting a potential NOAA CDR Science Strategy as an element of NOAA’s Climate Interventions Portfolio. NOAA Special Report. NOAA, Washington DC. DOI: 10.25923/gzke-8730 (Durability of sequestered carbon will depend on multiple variables and can vary greatly between individual ecosystems)
Challenges
- Accounting:
-
- Estimating future sequestration potentials or future rates of carbon sequestration is more difficult than estimating their current global extent and storage.
- While there is general scientific consensus that mangroves are net sinks of CO2[9]Rosentreter, Judith A., et al. “Coastal vegetation and estuaries are collectively a greenhouse gas sink.” Nature Climate Change, vol. 13, no. 6, 2023, pp. 579–587, https://doi.org/10.1038/s41558-023-01682-9. , there is growing research that indicates production of CH4 and N2O nonuniformly across habitats. In order to have accurate calculations of greenhouse gas budgets, these emissions will need to be included in the accounting[10]Collins, J. R., Boenish, R. E., Kleisner, K. M., Rader, D. N., Fujita, R. M., and Moritsch M. M. 2022. Coastal natural climate solutions: an assessment of scientific knowledge surrounding pathways for carbon dioxide removal and avoided emissions in nearshore blue carbon ecosystems. Environmental Defense Fund, New York, NY. www.edf.org/sites/default/files/2022-10/Coastal%20Natural%20Climate%20Solutions.pdf .
- Current estimates of sequestration potential do not account for the effects of climate change on the ecosystem (e.g., warming, sea level rise, coastal squeeze, increased tropical storms).
- Mangroves are inextricably connected to other marine ecosystems like seagrasses and coral reefs, complicating the definition of boundaries for estimating “additional” carbon sequestration and introducing other complications, such as figuring out how much carbon is autochthonous and how much is allochthonous. Additionally, many carbon markets currently require that all carbon be autochthonous to count toward credits or have poorly defined frameworks for whether to credit for allochthonous carbon that accumulates on site as a result of restoration or management actions.
- Restoration:
-
- There are high costs associated with restoration efforts[11]Vanderklift M, Steven A, Marcos-Martinez R, Gorman D (2018) Achieving carbon offsets through blue carbon: a review of needs and opportunities relevant to the Australian seafood industry, FRDC Final Report 2018/060. CSIRO Oceans and Atmosphere, Crawley, November 2018. .
- Mangrove ecosystems are heterogeneous across geographies, and this must be accounted for when assessing individual habitat potential for carbon sequestration. (e.g., findings from one location/ecosystem may not be directly applicable to other location/ecosystems
- There is work being done to use unmanned aerial systems (UAS) to assess mangrove estuaries on the Pacific Coast of Costa Rica[12]Yaney-Keller A, Santidrián Tomillo P, Marshall JM, Paladino FV (2019) Using Unmanned Aerial Systems (UAS) to assay mangrove estuaries on the Pacific coast of Costa Rica. PLoS ONE 14(6): e0217310. https://doi.org/10.1371/journal.pone.0217310 and in Malaysia[13]Otero, Viviana, et al. “Managing mangrove forests from the Sky: Forest Inventory using field data and Unmanned Aerial Vehicle (UAV) imagery in the Matang Mangrove Forest Reserve, peninsular Malaysia.” Forest Ecology and Management, vol. 411, 2018, pp. 35–45, https://doi.org/10.1016/j.foreco.2017.12.049. , enabling monitoring of difficult to access locations. This type of innovation may be important for monitoring and verification of restored mangrove forests.
Key Knowledge Gaps
- Quantitative understanding of the impacts of climate change on mangrove ecosystems and how these may affect restoration efforts.
- Incomplete understanding of greenhouse gas production and release (e.g., CH4 and N2O) by and from mangroves and how this impacts net carbon removal.
- Uncertainty around durability of carbon stored in mangroves and their sediments as well as carbon burial rates[14]Williamson P and Gattuso J-P (2022) Carbon Removal Using Coastal Blue Carbon Ecosystems Is Uncertain and Unreliable, With Questionable Climatic Cost-Effectiveness. Front. Clim. 4:853666. doi: 10.3389/fclim.2022.853666 .
First Order Priorities
*Adapted from the Environmental Defense Fund report on Coastal Natural Climate Solutions[15]Collins, J. R., Boenish, R. E., Kleisner, K. M., Rader, D. N., Fujita, R. M., and Moritsch M. M. 2022. Coastal natural climate solutions: an assessment of scientific knowledge surrounding pathways for carbon dioxide removal and avoided emissions in nearshore blue carbon ecosystems. Environmental Defense Fund, New York, NY. www.edf.org/sites/default/files/2022-10/Coastal%20Natural%20Climate%20Solutions.pdf
- Improve predictions of the effects of climate change on mangrove forests*
- Predictions needed include:
- Effects of increased tropical storm intensity and frequency on the destruction and degradation of mangrove ecosystems
- The timescales over which any previously sequestered carbon could be released to the atmosphere
- Understand/map the potential spatial extent of mangrove forests given climate change impacts on existing habitats and poleward migration of mangroves with future warming.
- Predictions needed include:
- Improved, spatially explicit modeling approaches to predict greenhouse gas fluxes and net carbon sequestration*
- Models should function across different mangrove ecosystems whose sediment dynamics cannot be resolved solely using imagery taken from space or aircraft.
- The development of carbon crediting projects requires we know not only how much carbon a mangrove ecosystem contains in its above and belowground biomass, but also the magnitude of various greenhouse gas fluxes from underlying soils or sediments at present and in the future.
- These models should account for future ecosystem state due to the interactive effects of climate change and predicted land-use changes, including the phenomenon of coastal squeeze, the prevention of expansion due to rural areas on one side and the ocean on the other.
- Create studies designed to better differentiate between carbon originating from within the system (autochthonous) and carbon originating from elsewhere (allochthonous)*
- The ability to distinguish among carbon sources is particularly critical since methodologies developed under the voluntary carbon market standards require deduction of allochthonous carbon from any claimed credit.
- Accelerate the development of technology and biotechnology that can help scale restoration efforts
- Optimize drones and autonomous robots for increased scalability of mangrove planting.
- Test biological engineering techniques to enhance carbon storage within mangrove forests.
Mechanism for CDR
- Restoring hydrology: Restoration of hydrology, through reversing drainage or removing tidal restrictions may assist in the recovery and expansion of existing mangrove sites. This path may be met with more resistance (especially political and social) than other types of restoration efforts.
- Planting mangrove seedlings: This is one of the most common restoration techniques, however, large-scale planting efforts have often failed, (many of these shortcomings a result of projects that do not adequately consider environmental and socioeconomic conditions) and smaller projects may not deliver carbon storage on climate-relevant scales.
-
- Research is being done on the efficacy of automated mangrove planting via drones,. This work is still in early stages but may provide lower cost options for planting efforts, especially in difficult to access locations.
- Restoration efforts should be de-risked in smaller projects before moving to larger projects. This will require investment in capacity-building in communities and institutions, and mechanisms to match restoration opportunities with prospective supporters and investors.
CDR Potential
Estimated Sequestration Potential: 0.028 – 0.172 Gt CO2e/year
Sequestration Durability: Decades -- 1,000 years (Durability of sequestered carbon will depend on multiple variables and can vary greatly between individual ecosystems)
Challenges
- Accounting:
-
- Estimating future sequestration potentials or future rates of carbon sequestration is more difficult than estimating their current global extent and storage.
- While there is general scientific consensus that mangroves are net sinks of CO2, there is growing research that indicates production of CH4 and N2O nonuniformly across habitats. In order to have accurate calculations of greenhouse gas budgets, these emissions will need to be included in the accounting.
- Current estimates of sequestration potential do not account for the effects of climate change on the ecosystem (e.g., warming, sea level rise, coastal squeeze, increased tropical storms).
- Mangroves are inextricably connected to other marine ecosystems like seagrasses and coral reefs, complicating the definition of boundaries for estimating “additional” carbon sequestration and introducing other complications, such as figuring out how much carbon is autochthonous and how much is allochthonous. Additionally, many carbon markets currently require that all carbon be autochthonous to count toward credits or have poorly defined frameworks for whether to credit for allochthonous carbon that accumulates on site as a result of restoration or management actions.
- Restoration:
-
- There are high costs associated with restoration efforts.
- Mangrove ecosystems are heterogeneous across geographies, and this must be accounted for when assessing individual habitat potential for carbon sequestration. (e.g., findings from one location/ecosystem may not be directly applicable to other location/ecosystems
- There is work being done to use unmanned aerial systems (UAS) to assess mangrove estuaries on the Pacific Coast of Costa Rica and in Malaysia, enabling monitoring of difficult to access locations. This type of innovation may be important for monitoring and verification of restored mangrove forests.
Key Knowledge Gaps
- Quantitative understanding of the impacts of climate change on mangrove ecosystems and how these may affect restoration efforts.
- Incomplete understanding of greenhouse gas production and release (e.g., CH4 and N2O) by and from mangroves and how this impacts net carbon removal.
- Uncertainty around durability of carbon stored in mangroves and their sediments as well as carbon burial rates.
First Order Priorities
*Adapted from the Environmental Defense Fund report on Coastal Natural Climate Solutions
- Improve predictions of the effects of climate change on mangrove forests*
- Predictions needed include:
- Effects of increased tropical storm intensity and frequency on the destruction and degradation of mangrove ecosystems
- The timescales over which any previously sequestered carbon could be released to the atmosphere
- Understand/map the potential spatial extent of mangrove forests given climate change impacts on existing habitats and poleward migration of mangroves with future warming.
- Predictions needed include:
- Improved, spatially explicit modeling approaches to predict greenhouse gas fluxes and net carbon sequestration*
- Models should function across different mangrove ecosystems whose sediment dynamics cannot be resolved solely using imagery taken from space or aircraft.
- The development of carbon crediting projects requires we know not only how much carbon a mangrove ecosystem contains in its above and belowground biomass, but also the magnitude of various greenhouse gas fluxes from underlying soils or sediments at present and in the future.
- These models should account for future ecosystem state due to the interactive effects of climate change and predicted land-use changes, including the phenomenon of coastal squeeze, the prevention of expansion due to rural areas on one side and the ocean on the other.
- Create studies designed to better differentiate between carbon originating from within the system (autochthonous) and carbon originating from elsewhere (allochthonous)*
- The ability to distinguish among carbon sources is particularly critical since methodologies developed under the voluntary carbon market standards require deduction of allochthonous carbon from any claimed credit.
- Accelerate the development of technology and biotechnology that can help scale restoration efforts
- Optimize drones and autonomous robots for increased scalability of mangrove planting.
- Test biological engineering techniques to enhance carbon storage within mangrove forests.
Mechanism for CDR
- Restoring hydrology: Restoration of hydrology, through reversing drainage or removing tidal restrictions may assist in the recovery and expansion of existing mangrove sites. This path may be met with more resistance (especially political and social) than other types of restoration efforts.
- Planting mangrove seedlings: This is one of the most common restoration techniques, however, large-scale planting efforts have often failed[1]Zimmer M, Ajonina GN, Amir AA, Cragg SM, Crooks S, Dahdouh-Guebas F, Duke NC, Fratini S, Friess DA, Helfer V, Huxham M, Kathiresan K, Kodikara KAS, Koedam N, Lee SY, Mangora MM, Primavera J, Satyanarayana B, Yong JWH and Wodehouse D (2022) When nature needs a helping hand: Different levels of human intervention for mangrove (re-)establishment. Front. For. Glob. Change 5:784322. doi: 10.3389/ffgc.2022.784322 , (many of these shortcomings a result of projects that do not adequately consider environmental and socioeconomic conditions[2]Lovelock CE,Barbier E,Duarte CM(2022) Tackling the mangrove restoration challenge. PLoS Biol20(10): e3001836. https://doi.org/10.1371/ journal.pbio.3001836 ) and smaller projects may not deliver carbon storage on climate-relevant scales[3]Lovelock CE, Barbier E, Duarte CM (2022) Tackling the mangrove restoration challenge. PLoS Biol 20(10): e3001836. https://doi.org/10.1371/ journal.pbio.3001836 .
-
- Research is being done on the efficacy of automated mangrove planting via drones[4]AlRaisi, A. A., et al. “Restoration technology hand in hand with nature-based solutions: ADNOC’s drone led Mangrove Restoration Project.” Day 1 Mon, October 02, 2023, 2023, https://doi.org/10.2118/215963-ms. ,[5]Ayub, Ayub Sugara, et al. “Mapping the potential of mangrove planting in the rehabilitation of coastal ecosystems using drone technology.” Journal of Sylva Indonesiana, vol. 6, no. 02, 2023, pp. 164–177, https://doi.org/10.32734/jsi.v6i02.10515. . This work is still in early stages but may provide lower cost options for planting efforts, especially in difficult to access locations.
- Restoration efforts should be de-risked in smaller projects before moving to larger projects. This will require investment in capacity-building in communities and institutions, and mechanisms to match restoration opportunities with prospective supporters and investors[6]Lovelock CE, Barbier E, Duarte CM (2022) Tackling the mangrove restoration challenge. PLoS Biol 20(10): e3001836. https://doi.org/10.1371/ journal.pbio.3001836 .
CDR Potential
Estimated Sequestration Potential: 0.028 – 0.172 Gt CO2e/year[7]Hoegh-Guldberg, O., Northrop, E. et al. 2023. "The ocean as a solution to climate change: Updated opportunities for action." Special Report. Washington, DC: World Resources Institute. Available online at https://oceanpanel.org/publication/ocean-solutions-to-climate-change
Sequestration Durability: Decades -- > 1,000 years[8]Cross, J.N., Sweeney, C., Jewett, E.B., Feely, R.A., McElhany, P., Carter, B., Stein, T., Kitch, G.D., and Gledhill, D.K., 2023. Strategy for NOAA Carbon Dioxide Removal Research: A white paper documenting a potential NOAA CDR Science Strategy as an element of NOAA’s Climate Interventions Portfolio. NOAA Special Report. NOAA, Washington DC. DOI: 10.25923/gzke-8730 (Durability of sequestered carbon will depend on multiple variables and can vary greatly between individual ecosystems)
Challenges
- Accounting:
-
- Estimating future sequestration potentials or future rates of carbon sequestration is more difficult than estimating their current global extent and storage.
- While there is general scientific consensus that mangroves are net sinks of CO2[9]Rosentreter, Judith A., et al. “Coastal vegetation and estuaries are collectively a greenhouse gas sink.” Nature Climate Change, vol. 13, no. 6, 2023, pp. 579–587, https://doi.org/10.1038/s41558-023-01682-9. , there is growing research that indicates production of CH4 and N2O nonuniformly across habitats. In order to have accurate calculations of greenhouse gas budgets, these emissions will need to be included in the accounting[10]Collins, J. R., Boenish, R. E., Kleisner, K. M., Rader, D. N., Fujita, R. M., and Moritsch M. M. 2022. Coastal natural climate solutions: an assessment of scientific knowledge surrounding pathways for carbon dioxide removal and avoided emissions in nearshore blue carbon ecosystems. Environmental Defense Fund, New York, NY. www.edf.org/sites/default/files/2022-10/Coastal%20Natural%20Climate%20Solutions.pdf .
- Current estimates of sequestration potential do not account for the effects of climate change on the ecosystem (e.g., warming, sea level rise, coastal squeeze, increased tropical storms).
- Mangroves are inextricably connected to other marine ecosystems like seagrasses and coral reefs, complicating the definition of boundaries for estimating “additional” carbon sequestration and introducing other complications, such as figuring out how much carbon is autochthonous and how much is allochthonous. Additionally, many carbon markets currently require that all carbon be autochthonous to count toward credits or have poorly defined frameworks for whether to credit for allochthonous carbon that accumulates on site as a result of restoration or management actions.
- Restoration:
-
- There are high costs associated with restoration efforts[11]Vanderklift M, Steven A, Marcos-Martinez R, Gorman D (2018) Achieving carbon offsets through blue carbon: a review of needs and opportunities relevant to the Australian seafood industry, FRDC Final Report 2018/060. CSIRO Oceans and Atmosphere, Crawley, November 2018. .
- Mangrove ecosystems are heterogeneous across geographies, and this must be accounted for when assessing individual habitat potential for carbon sequestration. (e.g., findings from one location/ecosystem may not be directly applicable to other location/ecosystems
- There is work being done to use unmanned aerial systems (UAS) to assess mangrove estuaries on the Pacific Coast of Costa Rica[12]Yaney-Keller A, Santidrián Tomillo P, Marshall JM, Paladino FV (2019) Using Unmanned Aerial Systems (UAS) to assay mangrove estuaries on the Pacific coast of Costa Rica. PLoS ONE 14(6): e0217310. https://doi.org/10.1371/journal.pone.0217310 and in Malaysia[13]Otero, Viviana, et al. “Managing mangrove forests from the Sky: Forest Inventory using field data and Unmanned Aerial Vehicle (UAV) imagery in the Matang Mangrove Forest Reserve, peninsular Malaysia.” Forest Ecology and Management, vol. 411, 2018, pp. 35–45, https://doi.org/10.1016/j.foreco.2017.12.049. , enabling monitoring of difficult to access locations. This type of innovation may be important for monitoring and verification of restored mangrove forests.
Key Knowledge Gaps
- Quantitative understanding of the impacts of climate change on mangrove ecosystems and how these may affect restoration efforts.
- Incomplete understanding of greenhouse gas production and release (e.g., CH4 and N2O) by and from mangroves and how this impacts net carbon removal.
- Uncertainty around durability of carbon stored in mangroves and their sediments as well as carbon burial rates[14]Williamson P and Gattuso J-P (2022) Carbon Removal Using Coastal Blue Carbon Ecosystems Is Uncertain and Unreliable, With Questionable Climatic Cost-Effectiveness. Front. Clim. 4:853666. doi: 10.3389/fclim.2022.853666 .
First Order Priorities
*Adapted from the Environmental Defense Fund report on Coastal Natural Climate Solutions[15]Collins, J. R., Boenish, R. E., Kleisner, K. M., Rader, D. N., Fujita, R. M., and Moritsch M. M. 2022. Coastal natural climate solutions: an assessment of scientific knowledge surrounding pathways for carbon dioxide removal and avoided emissions in nearshore blue carbon ecosystems. Environmental Defense Fund, New York, NY. www.edf.org/sites/default/files/2022-10/Coastal%20Natural%20Climate%20Solutions.pdf
- Improve predictions of the effects of climate change on mangrove forests*
- Predictions needed include:
- Effects of increased tropical storm intensity and frequency on the destruction and degradation of mangrove ecosystems
- The timescales over which any previously sequestered carbon could be released to the atmosphere
- Understand/map the potential spatial extent of mangrove forests given climate change impacts on existing habitats and poleward migration of mangroves with future warming.
- Predictions needed include:
- Improved, spatially explicit modeling approaches to predict greenhouse gas fluxes and net carbon sequestration*
- Models should function across different mangrove ecosystems whose sediment dynamics cannot be resolved solely using imagery taken from space or aircraft.
- The development of carbon crediting projects requires we know not only how much carbon a mangrove ecosystem contains in its above and belowground biomass, but also the magnitude of various greenhouse gas fluxes from underlying soils or sediments at present and in the future.
- These models should account for future ecosystem state due to the interactive effects of climate change and predicted land-use changes, including the phenomenon of coastal squeeze, the prevention of expansion due to rural areas on one side and the ocean on the other.
- Create studies designed to better differentiate between carbon originating from within the system (autochthonous) and carbon originating from elsewhere (allochthonous)*
- The ability to distinguish among carbon sources is particularly critical since methodologies developed under the voluntary carbon market standards require deduction of allochthonous carbon from any claimed credit.
- Accelerate the development of technology and biotechnology that can help scale restoration efforts
- Optimize drones and autonomous robots for increased scalability of mangrove planting.
- Test biological engineering techniques to enhance carbon storage within mangrove forests.
Mechanism for CDR
- Restoring hydrology: Restoration of hydrology, through reversing drainage or removing tidal restrictions may assist in the recovery and expansion of existing mangrove sites. This path may be met with more resistance (especially political and social) than other types of restoration efforts.
- Planting mangrove seedlings: This is one of the most common restoration techniques, however, large-scale planting efforts have often failed[1]Zimmer M, Ajonina GN, Amir AA, Cragg SM, Crooks S, Dahdouh-Guebas F, Duke NC, Fratini S, Friess DA, Helfer V, Huxham M, Kathiresan K, Kodikara KAS, Koedam N, Lee SY, Mangora MM, Primavera J, Satyanarayana B, Yong JWH and Wodehouse D (2022) When nature needs a helping hand: Different levels of human intervention for mangrove (re-)establishment. Front. For. Glob. Change 5:784322. doi: 10.3389/ffgc.2022.784322 , (many of these shortcomings a result of projects that do not adequately consider environmental and socioeconomic conditions[2]Lovelock CE,Barbier E,Duarte CM(2022) Tackling the mangrove restoration challenge. PLoS Biol20(10): e3001836. https://doi.org/10.1371/ journal.pbio.3001836 ) and smaller projects may not deliver carbon storage on climate-relevant scales[3]Lovelock CE, Barbier E, Duarte CM (2022) Tackling the mangrove restoration challenge. PLoS Biol 20(10): e3001836. https://doi.org/10.1371/ journal.pbio.3001836 .
-
- Research is being done on the efficacy of automated mangrove planting via drones[4]AlRaisi, A. A., et al. “Restoration technology hand in hand with nature-based solutions: ADNOC’s drone led Mangrove Restoration Project.” Day 1 Mon, October 02, 2023, 2023, https://doi.org/10.2118/215963-ms. ,[5]Ayub, Ayub Sugara, et al. “Mapping the potential of mangrove planting in the rehabilitation of coastal ecosystems using drone technology.” Journal of Sylva Indonesiana, vol. 6, no. 02, 2023, pp. 164–177, https://doi.org/10.32734/jsi.v6i02.10515. . This work is still in early stages but may provide lower cost options for planting efforts, especially in difficult to access locations.
- Restoration efforts should be de-risked in smaller projects before moving to larger projects. This will require investment in capacity-building in communities and institutions, and mechanisms to match restoration opportunities with prospective supporters and investors[6]Lovelock CE, Barbier E, Duarte CM (2022) Tackling the mangrove restoration challenge. PLoS Biol 20(10): e3001836. https://doi.org/10.1371/ journal.pbio.3001836 .
CDR Potential
Estimated Sequestration Potential: 0.028 – 0.172 Gt CO2e/year[7]Hoegh-Guldberg, O., Northrop, E. et al. 2023. "The ocean as a solution to climate change: Updated opportunities for action." Special Report. Washington, DC: World Resources Institute. Available online at https://oceanpanel.org/publication/ocean-solutions-to-climate-change
Sequestration Durability: Decades -- > 1,000 years[8]Cross, J.N., Sweeney, C., Jewett, E.B., Feely, R.A., McElhany, P., Carter, B., Stein, T., Kitch, G.D., and Gledhill, D.K., 2023. Strategy for NOAA Carbon Dioxide Removal Research: A white paper documenting a potential NOAA CDR Science Strategy as an element of NOAA’s Climate Interventions Portfolio. NOAA Special Report. NOAA, Washington DC. DOI: 10.25923/gzke-8730 (Durability of sequestered carbon will depend on multiple variables and can vary greatly between individual ecosystems)
Challenges
- Accounting:
-
- Estimating future sequestration potentials or future rates of carbon sequestration is more difficult than estimating their current global extent and storage.
- While there is general scientific consensus that mangroves are net sinks of CO2[9]Rosentreter, Judith A., et al. “Coastal vegetation and estuaries are collectively a greenhouse gas sink.” Nature Climate Change, vol. 13, no. 6, 2023, pp. 579–587, https://doi.org/10.1038/s41558-023-01682-9. , there is growing research that indicates production of CH4 and N2O nonuniformly across habitats. In order to have accurate calculations of greenhouse gas budgets, these emissions will need to be included in the accounting[10]Collins, J. R., Boenish, R. E., Kleisner, K. M., Rader, D. N., Fujita, R. M., and Moritsch M. M. 2022. Coastal natural climate solutions: an assessment of scientific knowledge surrounding pathways for carbon dioxide removal and avoided emissions in nearshore blue carbon ecosystems. Environmental Defense Fund, New York, NY. www.edf.org/sites/default/files/2022-10/Coastal%20Natural%20Climate%20Solutions.pdf .
- Current estimates of sequestration potential do not account for the effects of climate change on the ecosystem (e.g., warming, sea level rise, coastal squeeze, increased tropical storms).
- Mangroves are inextricably connected to other marine ecosystems like seagrasses and coral reefs, complicating the definition of boundaries for estimating “additional” carbon sequestration and introducing other complications, such as figuring out how much carbon is autochthonous and how much is allochthonous. Additionally, many carbon markets currently require that all carbon be autochthonous to count toward credits or have poorly defined frameworks for whether to credit for allochthonous carbon that accumulates on site as a result of restoration or management actions.
- Restoration:
-
- There are high costs associated with restoration efforts[11]Vanderklift M, Steven A, Marcos-Martinez R, Gorman D (2018) Achieving carbon offsets through blue carbon: a review of needs and opportunities relevant to the Australian seafood industry, FRDC Final Report 2018/060. CSIRO Oceans and Atmosphere, Crawley, November 2018. .
- Mangrove ecosystems are heterogeneous across geographies, and this must be accounted for when assessing individual habitat potential for carbon sequestration. (e.g., findings from one location/ecosystem may not be directly applicable to other location/ecosystems
- There is work being done to use unmanned aerial systems (UAS) to assess mangrove estuaries on the Pacific Coast of Costa Rica[12]Yaney-Keller A, Santidrián Tomillo P, Marshall JM, Paladino FV (2019) Using Unmanned Aerial Systems (UAS) to assay mangrove estuaries on the Pacific coast of Costa Rica. PLoS ONE 14(6): e0217310. https://doi.org/10.1371/journal.pone.0217310 and in Malaysia[13]Otero, Viviana, et al. “Managing mangrove forests from the Sky: Forest Inventory using field data and Unmanned Aerial Vehicle (UAV) imagery in the Matang Mangrove Forest Reserve, peninsular Malaysia.” Forest Ecology and Management, vol. 411, 2018, pp. 35–45, https://doi.org/10.1016/j.foreco.2017.12.049. , enabling monitoring of difficult to access locations. This type of innovation may be important for monitoring and verification of restored mangrove forests.
Key Knowledge Gaps
- Quantitative understanding of the impacts of climate change on mangrove ecosystems and how these may affect restoration efforts.
- Incomplete understanding of greenhouse gas production and release (e.g., CH4 and N2O) by and from mangroves and how this impacts net carbon removal.
- Uncertainty around durability of carbon stored in mangroves and their sediments as well as carbon burial rates[14]Williamson P and Gattuso J-P (2022) Carbon Removal Using Coastal Blue Carbon Ecosystems Is Uncertain and Unreliable, With Questionable Climatic Cost-Effectiveness. Front. Clim. 4:853666. doi: 10.3389/fclim.2022.853666 .
First Order Priorities
*Adapted from the Environmental Defense Fund report on Coastal Natural Climate Solutions[15]Collins, J. R., Boenish, R. E., Kleisner, K. M., Rader, D. N., Fujita, R. M., and Moritsch M. M. 2022. Coastal natural climate solutions: an assessment of scientific knowledge surrounding pathways for carbon dioxide removal and avoided emissions in nearshore blue carbon ecosystems. Environmental Defense Fund, New York, NY. www.edf.org/sites/default/files/2022-10/Coastal%20Natural%20Climate%20Solutions.pdf
- Improve predictions of the effects of climate change on mangrove forests*
- Predictions needed include:
- Effects of increased tropical storm intensity and frequency on the destruction and degradation of mangrove ecosystems
- The timescales over which any previously sequestered carbon could be released to the atmosphere
- Understand/map the potential spatial extent of mangrove forests given climate change impacts on existing habitats and poleward migration of mangroves with future warming.
- Predictions needed include:
- Improved, spatially explicit modeling approaches to predict greenhouse gas fluxes and net carbon sequestration*
- Models should function across different mangrove ecosystems whose sediment dynamics cannot be resolved solely using imagery taken from space or aircraft.
- The development of carbon crediting projects requires we know not only how much carbon a mangrove ecosystem contains in its above and belowground biomass, but also the magnitude of various greenhouse gas fluxes from underlying soils or sediments at present and in the future.
- These models should account for future ecosystem state due to the interactive effects of climate change and predicted land-use changes, including the phenomenon of coastal squeeze, the prevention of expansion due to rural areas on one side and the ocean on the other.
- Create studies designed to better differentiate between carbon originating from within the system (autochthonous) and carbon originating from elsewhere (allochthonous)*
- The ability to distinguish among carbon sources is particularly critical since methodologies developed under the voluntary carbon market standards require deduction of allochthonous carbon from any claimed credit.
- Accelerate the development of technology and biotechnology that can help scale restoration efforts
- Optimize drones and autonomous robots for increased scalability of mangrove planting.
- Test biological engineering techniques to enhance carbon storage within mangrove forests.
Mechanism for CDR
- Restoring hydrology: Restoration of hydrology, through reversing drainage or removing tidal restrictions may assist in the recovery and expansion of existing mangrove sites. This path may be met with more resistance (especially political and social) than other types of restoration efforts.
- Planting mangrove seedlings: This is one of the most common restoration techniques, however, large-scale planting efforts have often failed[1] , (many of these shortcomings a result of projects that do not adequately consider environmental and socioeconomic conditions[2] ) and smaller projects may not deliver carbon storage on climate-relevant scales[3] .
- Restoration efforts should be de-risked in smaller projects before moving to larger projects. This will require investment in capacity-building in communities and institutions, and mechanisms to match restoration opportunities with prospective supporters and investors[6] .
CDR Potential
Estimated Sequestration Potential: 0.028 – 0.172 Gt CO2e/year[7]
Sequestration Durability: Decades -- > 1,000 years[8] (Durability of sequestered carbon will depend on multiple variables and can vary greatly between individual ecosystems)
Challenges
- Accounting:
-
- Estimating future sequestration potentials or future rates of carbon sequestration is more difficult than estimating their current global extent and storage.
- While there is general scientific consensus that mangroves are net sinks of CO2[9] , there is growing research that indicates production of CH4 and N2O nonuniformly across habitats. In order to have accurate calculations of greenhouse gas budgets, these emissions will need to be included in the accounting[10] .
- Current estimates of sequestration potential do not account for the effects of climate change on the ecosystem (e.g., warming, sea level rise, coastal squeeze, increased tropical storms).
- Mangroves are inextricably connected to other marine ecosystems like seagrasses and coral reefs, complicating the definition of boundaries for estimating “additional” carbon sequestration and introducing other complications, such as figuring out how much carbon is autochthonous and how much is allochthonous. Additionally, many carbon markets currently require that all carbon be autochthonous to count toward credits or have poorly defined frameworks for whether to credit for allochthonous carbon that accumulates on site as a result of restoration or management actions.
- Restoration:
-
- There are high costs associated with restoration efforts[11] .
- Mangrove ecosystems are heterogeneous across geographies, and this must be accounted for when assessing individual habitat potential for carbon sequestration. (e.g., findings from one location/ecosystem may not be directly applicable to other location/ecosystems
- There is work being done to use unmanned aerial systems (UAS) to assess mangrove estuaries on the Pacific Coast of Costa Rica[12] and in Malaysia[13] , enabling monitoring of difficult to access locations. This type of innovation may be important for monitoring and verification of restored mangrove forests.
Key Knowledge Gaps
- Quantitative understanding of the impacts of climate change on mangrove ecosystems and how these may affect restoration efforts.
- Incomplete understanding of greenhouse gas production and release (e.g., CH4 and N2O) by and from mangroves and how this impacts net carbon removal.
- Uncertainty around durability of carbon stored in mangroves and their sediments as well as carbon burial rates[14] .
First Order Priorities
*Adapted from the Environmental Defense Fund report on Coastal Natural Climate Solutions[15]
- Improve predictions of the effects of climate change on mangrove forests*
- Predictions needed include:
- Effects of increased tropical storm intensity and frequency on the destruction and degradation of mangrove ecosystems
- The timescales over which any previously sequestered carbon could be released to the atmosphere
- Understand/map the potential spatial extent of mangrove forests given climate change impacts on existing habitats and poleward migration of mangroves with future warming.
- Predictions needed include:
- Improved, spatially explicit modeling approaches to predict greenhouse gas fluxes and net carbon sequestration*
- Models should function across different mangrove ecosystems whose sediment dynamics cannot be resolved solely using imagery taken from space or aircraft.
- The development of carbon crediting projects requires we know not only how much carbon a mangrove ecosystem contains in its above and belowground biomass, but also the magnitude of various greenhouse gas fluxes from underlying soils or sediments at present and in the future.
- These models should account for future ecosystem state due to the interactive effects of climate change and predicted land-use changes, including the phenomenon of coastal squeeze, the prevention of expansion due to rural areas on one side and the ocean on the other.
- Create studies designed to better differentiate between carbon originating from within the system (autochthonous) and carbon originating from elsewhere (allochthonous)*
- The ability to distinguish among carbon sources is particularly critical since methodologies developed under the voluntary carbon market standards require deduction of allochthonous carbon from any claimed credit.
- Accelerate the development of technology and biotechnology that can help scale restoration efforts
- Optimize drones and autonomous robots for increased scalability of mangrove planting.
- Test biological engineering techniques to enhance carbon storage within mangrove forests.
Salt Marshes
Mechanism for CDR
- Most restoration efforts include recovery of tidal exchange (e.g., restoring hydrology and tidal flow), managed realignment, and sediment level amendment (Billah et al., 2022). Efforts can also include removal of non-native vegetation and/or replanting of native vegetation.
- For an in-depth look at salt marsh restoration efforts to date, refer to Adam 2019 “Salt Marsh Restoration” (Adam, 2019)
CDR Potential
Estimated Sequestration Potential: 0.004 – 0.015 Gt CO2e/year (Hoegh-Guldberg et al., 2023)
Sequestration Durability: Decades — 1,000 years (Cross et al., 2023) (Durability of sequestered carbon will depend on multiple variables and can vary greatly between individual ecosystems)
Challenges
- Little potential for expansion of current habitats due to extensive land conversion.
- Gaining access to suitable land for restoration projects may be difficult and expensive.
- Determining baseline historical extent for setting restoration objectives.
Key Knowledge Gaps
- Need accurate predictions of carbon sequestration which requires integrated modeling of ecological impacts, land-use, and climate effects (made more challenging by high variability in carbon burial rates (Williamson & Gattuso, 2022)).
- Discerning between competing feedbacks associated with climate and sea level rise, which can have both positive and negative impacts on ecosystems.
- Measurement of greenhouse gases other than CO2 (e.g., CH4 and N2O) that are produced and their significance to net sequestration.
- What is the fate of carbon produced outside of marshes and trapped in them?
- Total global areal existing extent remains poorly mapped, especially at high latitudes.
First-Order Priorities
*Adapted from the Environmental Defense Fund report on Coastal Natural Climate Solutions (Collins et al., 2022)
- Conduct research to better understand and characterize the multiple carbon fluxes within salt marsh systems
- Improve predictions of carbon sequestered by salt marshes through modeling*
- Better knowledge of the relative contributions of carbon produced outside of (allochthonous) and within (autochthonous) marshes to salt marsh organic carbon pools, and the labilities of the carbon derived from these two sources*
- Investigate and characterize climate impacts, both positive and negative, on salt marsh systems*
- Sea level rise, warming, and increased coastal storm frequency and severity all need further investigation.
- Measure non-CO2 greenhouse gases that are produced and compare with sequestration capabilities to better understand the net sequestration of salt marshes*
- Better global mapping of the current extent of salt marshes, particularly at high latitudes, as well as habitat-suitability mapping/analysis*
Mechanism for CDR
- Most restoration efforts include recovery of tidal exchange (e.g., restoring hydrology and tidal flow), managed realignment, and sediment level amendment (Billah et al., 2022). Efforts can also include removal of non-native vegetation and/or replanting of native vegetation.
- For an in-depth look at salt marsh restoration efforts to date, refer to Adam 2019 “Salt Marsh Restoration” (Adam, 2019)
CDR Potential
Estimated Sequestration Potential: 0.004 – 0.015 Gt CO2e/year (Hoegh-Guldberg et al., 2023)
Sequestration Durability: Decades -- 1,000 years (Cross et al., 2023) (Durability of sequestered carbon will depend on multiple variables and can vary greatly between individual ecosystems)
Challenges
- Little potential for expansion of current habitats due to extensive land conversion.
- Gaining access to suitable land for restoration projects may be difficult and expensive.
- Determining baseline historical extent for setting restoration objectives.
Key Knowledge Gaps
- Need accurate predictions of carbon sequestration which requires integrated modeling of ecological impacts, land-use, and climate effects (made more challenging by high variability in carbon burial rates (Williamson & Gattuso, 2022)).
- Discerning between competing feedbacks associated with climate and sea level rise, which can have both positive and negative impacts on ecosystems.
- Measurement of greenhouse gases other than CO2 (e.g., CH4 and N2O) that are produced and their significance to net sequestration.
- What is the fate of carbon produced outside of marshes and trapped in them?
- Total global areal existing extent remains poorly mapped, especially at high latitudes.
First-Order Priorities
*Adapted from the Environmental Defense Fund report on Coastal Natural Climate Solutions (Collins et al., 2022)
- Conduct research to better understand and characterize the multiple carbon fluxes within salt marsh systems
- Improve predictions of carbon sequestered by salt marshes through modeling*
- Better knowledge of the relative contributions of carbon produced outside of (allochthonous) and within (autochthonous) marshes to salt marsh organic carbon pools, and the labilities of the carbon derived from these two sources*
- Investigate and characterize climate impacts, both positive and negative, on salt marsh systems*
- Sea level rise, warming, and increased coastal storm frequency and severity all need further investigation.
- Measure non-CO2 greenhouse gases that are produced and compare with sequestration capabilities to better understand the net sequestration of salt marshes*
- Better global mapping of the current extent of salt marshes, particularly at high latitudes, as well as habitat-suitability mapping/analysis*
Mechanism for CDR
- Most restoration efforts include recovery of tidal exchange (e.g., restoring hydrology and tidal flow), managed realignment, and sediment level amendment. Efforts can also include removal of non-native vegetation and/or replanting of native vegetation.
- For an in-depth look at salt marsh restoration efforts to date, refer to Adam 2019 “Salt Marsh Restoration”
CDR Potential
Estimated Sequestration Potential: 0.004 – 0.015 Gt CO2e/year
Sequestration Durability: Decades -- 1,000 years (Durability of sequestered carbon will depend on multiple variables and can vary greatly between individual ecosystems)
Challenges
- Little potential for expansion of current habitats due to extensive land conversion.
- Gaining access to suitable land for restoration projects may be difficult and expensive.
- Determining baseline historical extent for setting restoration objectives.
Key Knowledge Gaps
- Need accurate predictions of carbon sequestration which requires integrated modeling of ecological impacts, land-use, and climate effects (made more challenging by high variability in carbon burial rates).
- Discerning between competing feedbacks associated with climate and sea level rise, which can have both positive and negative impacts on ecosystems.
- Measurement of greenhouse gases other than CO2 (e.g., CH4 and N2O) that are produced and their significance to net sequestration.
- What is the fate of carbon produced outside of marshes and trapped in them?
- Total global areal existing extent remains poorly mapped, especially at high latitudes.
First-Order Priorities
*Adapted from the Environmental Defense Fund report on Coastal Natural Climate Solutions
- Conduct research to better understand and characterize the multiple carbon fluxes within salt marsh systems
- Improve predictions of carbon sequestered by salt marshes through modeling*
- Better knowledge of the relative contributions of carbon produced outside of (allochthonous) and within (autochthonous) marshes to salt marsh organic carbon pools, and the labilities of the carbon derived from these two sources*
- Investigate and characterize climate impacts, both positive and negative, on salt marsh systems*
- Sea level rise, warming, and increased coastal storm frequency and severity all need further investigation.
- Measure non-CO2 greenhouse gases that are produced and compare with sequestration capabilities to better understand the net sequestration of salt marshes*
- Better global mapping of the current extent of salt marshes, particularly at high latitudes, as well as habitat-suitability mapping/analysis*
Mechanism for CDR
- Most restoration efforts include recovery of tidal exchange (e.g., restoring hydrology and tidal flow), managed realignment, and sediment level amendment[1]Billah, M., Bhuiyan, M.A., Islam, M.A. et al. Salt marsh restoration: an overview of techniques and success indicators. Environ Sci Pollut Res 29, 15347–15363 (2022). https://doi.org/10.1007/s11356-021-18305-5 . Efforts can also include removal of non-native vegetation and/or replanting of native vegetation.
- For an in-depth look at salt marsh restoration efforts to date, refer to Adam 2019 “Salt Marsh Restoration”[2]Adam, Paul. “Salt Marsh Restoration.” Coastal Wetlands, 2019, pp. 817–861, https://doi.org/10.1016/b978-0-444-63893-9.00023-x.
CDR Potential
Estimated Sequestration Potential: 0.004 – 0.015 Gt CO2e/year[3]Hoegh-Guldberg, O., Northrop, E. et al. 2023. "The ocean as a solution to climate change: Updated opportunities for action." Special Report. Washington, DC: World Resources Institute. Available online at https://oceanpanel.org/publication/ocean-solutions-to-climate-change
Sequestration Durability: Decades -- 1,000 years[4]Cross, J.N., Sweeney, C., Jewett, E.B., Feely, R.A., McElhany, P., Carter, B., Stein, T., Kitch, G.D., and Gledhill, D.K., 2023. Strategy for NOAA Carbon Dioxide Removal Research: A white paper documenting a potential NOAA CDR Science Strategy as an element of NOAA’s Climate Interventions Portfolio. NOAA Special Report. NOAA, Washington DC. DOI: 10.25923/gzke-8730 (Durability of sequestered carbon will depend on multiple variables and can vary greatly between individual ecosystems)
Challenges
- Little potential for expansion of current habitats due to extensive land conversion.
- Gaining access to suitable land for restoration projects may be difficult and expensive.
- Determining baseline historical extent for setting restoration objectives.
Key Knowledge Gaps
- Need accurate predictions of carbon sequestration which requires integrated modeling of ecological impacts, land-use, and climate effects (made more challenging by high variability in carbon burial rates[5]Williamson P and Gattuso J-P (2022) Carbon Removal Using Coastal Blue Carbon Ecosystems Is Uncertain and Unreliable, With Questionable Climatic Cost-Effectiveness. Front. Clim. 4:853666. doi: 10.3389/fclim.2022.853666 ).
- Discerning between competing feedbacks associated with climate and sea level rise, which can have both positive and negative impacts on ecosystems.
- Measurement of greenhouse gases other than CO2 (e.g., CH4 and N2O) that are produced and their significance to net sequestration.
- What is the fate of carbon produced outside of marshes and trapped in them?
- Total global areal existing extent remains poorly mapped, especially at high latitudes.
First-Order Priorities
*Adapted from the Environmental Defense Fund report on Coastal Natural Climate Solutions[6]Collins, J. R., Boenish, R. E., Kleisner, K. M., Rader, D. N., Fujita, R. M., and Moritsch M. M. 2022. Coastal natural climate solutions: an assessment of scientific knowledge surrounding pathways for carbon dioxide removal and avoided emissions in nearshore blue carbon ecosystems. Environmental Defense Fund, New York, NY. https://www.edf.org/sites/default/files/2022-10/Coastal%20Natural%20Climate%20Solutions.pdf
- Conduct research to better understand and characterize the multiple carbon fluxes within salt marsh systems
- Improve predictions of carbon sequestered by salt marshes through modeling*
- Better knowledge of the relative contributions of carbon produced outside of (allochthonous) and within (autochthonous) marshes to salt marsh organic carbon pools, and the labilities of the carbon derived from these two sources*
- Investigate and characterize climate impacts, both positive and negative, on salt marsh systems*
- Sea level rise, warming, and increased coastal storm frequency and severity all need further investigation.
- Measure non-CO2 greenhouse gases that are produced and compare with sequestration capabilities to better understand the net sequestration of salt marshes*
- Better global mapping of the current extent of salt marshes, particularly at high latitudes, as well as habitat-suitability mapping/analysis*
Mechanism for CDR
- Most restoration efforts include recovery of tidal exchange (e.g., restoring hydrology and tidal flow), managed realignment, and sediment level amendment[1]Billah, M., Bhuiyan, M.A., Islam, M.A. et al. Salt marsh restoration: an overview of techniques and success indicators. Environ Sci Pollut Res 29, 15347–15363 (2022). https://doi.org/10.1007/s11356-021-18305-5 . Efforts can also include removal of non-native vegetation and/or replanting of native vegetation.
- For an in-depth look at salt marsh restoration efforts to date, refer to Adam 2019 “Salt Marsh Restoration”[2]Adam, Paul. “Salt Marsh Restoration.” Coastal Wetlands, 2019, pp. 817–861, https://doi.org/10.1016/b978-0-444-63893-9.00023-x.
CDR Potential
Estimated Sequestration Potential: 0.004 – 0.015 Gt CO2e/year[3]Hoegh-Guldberg, O., Northrop, E. et al. 2023. "The ocean as a solution to climate change: Updated opportunities for action." Special Report. Washington, DC: World Resources Institute. Available online at https://oceanpanel.org/publication/ocean-solutions-to-climate-change
Sequestration Durability: Decades -- 1,000 years[4]Cross, J.N., Sweeney, C., Jewett, E.B., Feely, R.A., McElhany, P., Carter, B., Stein, T., Kitch, G.D., and Gledhill, D.K., 2023. Strategy for NOAA Carbon Dioxide Removal Research: A white paper documenting a potential NOAA CDR Science Strategy as an element of NOAA’s Climate Interventions Portfolio. NOAA Special Report. NOAA, Washington DC. DOI: 10.25923/gzke-8730 (Durability of sequestered carbon will depend on multiple variables and can vary greatly between individual ecosystems)
Challenges
- Little potential for expansion of current habitats due to extensive land conversion.
- Gaining access to suitable land for restoration projects may be difficult and expensive.
- Determining baseline historical extent for setting restoration objectives.
Key Knowledge Gaps
- Need accurate predictions of carbon sequestration which requires integrated modeling of ecological impacts, land-use, and climate effects (made more challenging by high variability in carbon burial rates[5]Williamson P and Gattuso J-P (2022) Carbon Removal Using Coastal Blue Carbon Ecosystems Is Uncertain and Unreliable, With Questionable Climatic Cost-Effectiveness. Front. Clim. 4:853666. doi: 10.3389/fclim.2022.853666 ).
- Discerning between competing feedbacks associated with climate and sea level rise, which can have both positive and negative impacts on ecosystems.
- Measurement of greenhouse gases other than CO2 (e.g., CH4 and N2O) that are produced and their significance to net sequestration.
- What is the fate of carbon produced outside of marshes and trapped in them?
- Total global areal existing extent remains poorly mapped, especially at high latitudes.
First Order Priorities
*Adapted from the Environmental Defense Fund report on Coastal Natural Climate Solutions[6]Collins, J. R., Boenish, R. E., Kleisner, K. M., Rader, D. N., Fujita, R. M., and Moritsch M. M. 2022. Coastal natural climate solutions: an assessment of scientific knowledge surrounding pathways for carbon dioxide removal and avoided emissions in nearshore blue carbon ecosystems. Environmental Defense Fund, New York, NY. www.edf.org/sites/default/files/2022-10/Coastal%20Natural%20Climate%20Solutions.pdf
- Conduct research to better understand and characterize the multiple carbon fluxes within salt marsh systems
- Improve predictions of carbon sequestered by salt marshes through modeling*
- Better knowledge of the relative contributions of carbon produced outside of (allochthonous) and within (autochthonous) marshes to salt marsh organic carbon pools, and the labilities of the carbon derived from these two sources*
- Investigate and characterize climate impacts, both positive and negative, on salt marsh systems*
- Sea level rise, warming, and increased coastal storm frequency and severity all need further investigation.
- Measure non-CO2 greenhouse gases that are produced and compare with sequestration capabilities to better understand the net sequestration of salt marshes*
- Better global mapping of the current extent of salt marshes, particularly at high latitudes, as well as habitat-suitability mapping/analysis*
Mechanism for CDR
- Most restoration efforts include recovery of tidal exchange (e.g., restoring hydrology and tidal flow), managed realignment, and sediment level amendment[1]Billah, M., Bhuiyan, M.A., Islam, M.A. et al. Salt marsh restoration: an overview of techniques and success indicators. Environ Sci Pollut Res 29, 15347–15363 (2022). https://doi.org/10.1007/s11356-021-18305-5 . Efforts can also include removal of non-native vegetation and/or replanting of native vegetation.
- For an in-depth look at salt marsh restoration efforts to date, refer to Adam 2019 “Salt Marsh Restoration”[2]Adam, Paul. “Salt Marsh Restoration.” Coastal Wetlands, 2019, pp. 817–861, https://doi.org/10.1016/b978-0-444-63893-9.00023-x.
CDR Potential
Estimated Sequestration Potential: 0.004 – 0.015 Gt CO2e/year[3]Hoegh-Guldberg, O., Northrop, E. et al. 2023. "The ocean as a solution to climate change: Updated opportunities for action." Special Report. Washington, DC: World Resources Institute. Available online at https://oceanpanel.org/publication/ocean-solutions-to-climate-change
Sequestration Durability: Decades -- 1,000 years[4]Cross, J.N., Sweeney, C., Jewett, E.B., Feely, R.A., McElhany, P., Carter, B., Stein, T., Kitch, G.D., and Gledhill, D.K., 2023. Strategy for NOAA Carbon Dioxide Removal Research: A white paper documenting a potential NOAA CDR Science Strategy as an element of NOAA’s Climate Interventions Portfolio. NOAA Special Report. NOAA, Washington DC. DOI: 10.25923/gzke-8730 (Durability of sequestered carbon will depend on multiple variables and can vary greatly between individual ecosystems)
Challenges
- Little potential for expansion of current habitats due to extensive land conversion.
- Gaining access to suitable land for restoration projects may be difficult and expensive.
- Determining baseline historical extent for setting restoration objectives.
Key Knowledge Gaps
- Need accurate predictions of carbon sequestration which requires integrated modeling of ecological impacts, land-use, and climate effects (made more challenging by high variability in carbon burial rates[5]Williamson P and Gattuso J-P (2022) Carbon Removal Using Coastal Blue Carbon Ecosystems Is Uncertain and Unreliable, With Questionable Climatic Cost-Effectiveness. Front. Clim. 4:853666. doi: 10.3389/fclim.2022.853666 ).
- Discerning between competing feedbacks associated with climate and sea level rise, which can have both positive and negative impacts on ecosystems.
- Measurement of greenhouse gases other than CO2 (e.g., CH4 and N2O) that are produced and their significance to net sequestration.
- What is the fate of carbon produced outside of marshes and trapped in them?
- Total global areal existing extent remains poorly mapped, especially at high latitudes.
First Order Priorities
*Adapted from the Environmental Defense Fund report on Coastal Natural Climate Solutions[6]Collins, J. R., Boenish, R. E., Kleisner, K. M., Rader, D. N., Fujita, R. M., and Moritsch M. M. 2022. Coastal natural climate solutions: an assessment of scientific knowledge surrounding pathways for carbon dioxide removal and avoided emissions in nearshore blue carbon ecosystems. Environmental Defense Fund, New York, NY. www.edf.org/sites/default/files/2022-10/Coastal%20Natural%20Climate%20Solutions.pdf
- Conduct research to better understand and characterize the multiple carbon fluxes within salt marsh systems
- Improve predictions of carbon sequestered by salt marshes through modeling*
- Better knowledge of the relative contributions of carbon produced outside of (allochthonous) and within (autochthonous) marshes to salt marsh organic carbon pools, and the labilities of the carbon derived from these two sources*
- Investigate and characterize climate impacts, both positive and negative, on salt marsh systems*
- Sea level rise, warming, and increased coastal storm frequency and severity all need further investigation.
- Measure non-CO2 greenhouse gases that are produced and compare with sequestration capabilities to better understand the net sequestration of salt marshes*
- Better global mapping of the current extent of salt marshes, particularly at high latitudes, as well as habitat-suitability mapping/analysis*
Mechanism for CDR
- Most restoration efforts include recovery of tidal exchange (e.g., restoring hydrology and tidal flow), managed realignment, and sediment level amendment[1]Billah, M., Bhuiyan, M.A., Islam, M.A. et al. Salt marsh restoration: an overview of techniques and success indicators. Environ Sci Pollut Res 29, 15347–15363 (2022). https://doi.org/10.1007/s11356-021-18305-5 . Efforts can also include removal of non-native vegetation and/or replanting of native vegetation.
- For an in-depth look at salt marsh restoration efforts to date, refer to Adam 2019 “Salt Marsh Restoration”[2]Adam, Paul. “Salt Marsh Restoration.” Coastal Wetlands, 2019, pp. 817–861, https://doi.org/10.1016/b978-0-444-63893-9.00023-x.
CDR Potential
Estimated Sequestration Potential: 0.004 – 0.015 Gt CO2e/year[3]Hoegh-Guldberg, O., Northrop, E. et al. 2023. "The ocean as a solution to climate change: Updated opportunities for action." Special Report. Washington, DC: World Resources Institute. Available online at https://oceanpanel.org/publication/ocean-solutions-to-climate-change
Sequestration Durability: Decades -- 1,000 years[4]Cross, J.N., Sweeney, C., Jewett, E.B., Feely, R.A., McElhany, P., Carter, B., Stein, T., Kitch, G.D., and Gledhill, D.K., 2023. Strategy for NOAA Carbon Dioxide Removal Research: A white paper documenting a potential NOAA CDR Science Strategy as an element of NOAA’s Climate Interventions Portfolio. NOAA Special Report. NOAA, Washington DC. DOI: 10.25923/gzke-8730 (Durability of sequestered carbon will depend on multiple variables and can vary greatly between individual ecosystems)
Challenges
- Little potential for expansion of current habitats due to extensive land conversion.
- Gaining access to suitable land for restoration projects may be difficult and expensive.
- Determining baseline historical extent for setting restoration objectives.
Key Knowledge Gaps
- Need accurate predictions of carbon sequestration which requires integrated modeling of ecological impacts, land-use, and climate effects (made more challenging by high variability in carbon burial rates[5]Williamson P and Gattuso J-P (2022) Carbon Removal Using Coastal Blue Carbon Ecosystems Is Uncertain and Unreliable, With Questionable Climatic Cost-Effectiveness. Front. Clim. 4:853666. doi: 10.3389/fclim.2022.853666 ).
- Discerning between competing feedbacks associated with climate and sea level rise, which can have both positive and negative impacts on ecosystems.
- Measurement of greenhouse gases other than CO2 (e.g., CH4 and N2O) that are produced and their significance to net sequestration.
- What is the fate of carbon produced outside of marshes and trapped in them?
- Total global areal existing extent remains poorly mapped, especially at high latitudes.
First Order Priorities
*Adapted from the Environmental Defense Fund report on Coastal Natural Climate Solutions[6]Collins, J. R., Boenish, R. E., Kleisner, K. M., Rader, D. N., Fujita, R. M., and Moritsch M. M. 2022. Coastal natural climate solutions: an assessment of scientific knowledge surrounding pathways for carbon dioxide removal and avoided emissions in nearshore blue carbon ecosystems. Environmental Defense Fund, New York, NY. www.edf.org/sites/default/files/2022-10/Coastal%20Natural%20Climate%20Solutions.pdf
- Conduct research to better understand and characterize the multiple carbon fluxes within salt marsh systems
- Improve predictions of carbon sequestered by salt marshes through modeling*
- Better knowledge of the relative contributions of carbon produced outside of (allochthonous) and within (autochthonous) marshes to salt marsh organic carbon pools, and the labilities of the carbon derived from these two sources*
- Investigate and characterize climate impacts, both positive and negative, on salt marsh systems*
- Sea level rise, warming, and increased coastal storm frequency and severity all need further investigation.
- Measure non-CO2 greenhouse gases that are produced and compare with sequestration capabilities to better understand the net sequestration of salt marshes*
- Better global mapping of the current extent of salt marshes, particularly at high latitudes, as well as habitat-suitability mapping/analysis*
Mechanism for CDR
- Most restoration efforts include recovery of tidal exchange (e.g., restoring hydrology and tidal flow), managed realignment, and sediment level amendment[1]Billah, M., Bhuiyan, M.A., Islam, M.A. et al. Salt marsh restoration: an overview of techniques and success indicators. Environ Sci Pollut Res 29, 15347–15363 (2022). https://doi.org/10.1007/s11356-021-18305-5 . Efforts can also include removal of non-native vegetation and/or replanting of native vegetation.
- For an in-depth look at salt marsh restoration efforts to date, refer to Adam 2019 “Salt Marsh Restoration”[2]Adam, Paul. “Salt Marsh Restoration.” Coastal Wetlands, 2019, pp. 817–861, https://doi.org/10.1016/b978-0-444-63893-9.00023-x.
CDR Potential
Estimated Sequestration Potential: 0.004 – 0.015 Gt CO2e/year[3]Hoegh-Guldberg, O., Northrop, E. et al. 2023. "The ocean as a solution to climate change: Updated opportunities for action." Special Report. Washington, DC: World Resources Institute. Available online at https://oceanpanel.org/publication/ocean-solutions-to-climate-change
Sequestration Durability: Decades -- > 1,000 years[4]Cross, J.N., Sweeney, C., Jewett, E.B., Feely, R.A., McElhany, P., Carter, B., Stein, T., Kitch, G.D., and Gledhill, D.K., 2023. Strategy for NOAA Carbon Dioxide Removal Research: A white paper documenting a potential NOAA CDR Science Strategy as an element of NOAA’s Climate Interventions Portfolio. NOAA Special Report. NOAA, Washington DC. DOI: 10.25923/gzke-8730 (Durability of sequestered carbon will depend on multiple variables and can vary greatly between individual ecosystems)
Challenges
- Little potential for expansion of current habitats due to extensive land conversion.
- Gaining access to suitable land for restoration projects may be difficult and expensive.
- Determining baseline historical extent for setting restoration objectives.
Key Knowledge Gaps
- Need accurate predictions of carbon sequestration which requires integrated modeling of ecological impacts, land-use, and climate effects (made more challenging by high variability in carbon burial rates[5]Williamson P and Gattuso J-P (2022) Carbon Removal Using Coastal Blue Carbon Ecosystems Is Uncertain and Unreliable, With Questionable Climatic Cost-Effectiveness. Front. Clim. 4:853666. doi: 10.3389/fclim.2022.853666 ).
- Discerning between competing feedbacks associated with climate and sea level rise, which can have both positive and negative impacts on ecosystems.
- Measurement of greenhouse gases other than CO2 (e.g., CH4 and N2O) that are produced and their significance to net sequestration.
- What is the fate of carbon produced outside of marshes and trapped in them?
- Total global areal existing extent remains poorly mapped, especially at high latitudes.
First Order Priorities
*Adapted from the Environmental Defense Fund report on Coastal Natural Climate Solutions[6]Collins, J. R., Boenish, R. E., Kleisner, K. M., Rader, D. N., Fujita, R. M., and Moritsch M. M. 2022. Coastal natural climate solutions: an assessment of scientific knowledge surrounding pathways for carbon dioxide removal and avoided emissions in nearshore blue carbon ecosystems. Environmental Defense Fund, New York, NY. www.edf.org/sites/default/files/2022-10/Coastal%20Natural%20Climate%20Solutions.pdf
- Conduct research to better understand and characterize the multiple carbon fluxes within salt marsh systems
- Improve predictions of carbon sequestered by salt marshes through modeling*
- Better knowledge of the relative contributions of carbon produced outside of (allochthonous) and within (autochthonous) marshes to salt marsh organic carbon pools, and the labilities of the carbon derived from these two sources*
- Investigate and characterize climate impacts, both positive and negative, on salt marsh systems*
- Sea level rise, warming, and increased coastal storm frequency and severity all need further investigation.
- Measure non-CO2 greenhouse gases that are produced and compare with sequestration capabilities to better understand the net sequestration of salt marshes*
- Better global mapping of the current extent of salt marshes, particularly at high latitudes, as well as habitat-suitability mapping/analysis*
Mechanism for CDR
- Most restoration efforts include recovery of tidal exchange (e.g., restoring hydrology and tidal flow), managed realignment, and sediment level amendment[1]Billah, M., Bhuiyan, M.A., Islam, M.A. et al. Salt marsh restoration: an overview of techniques and success indicators. Environ Sci Pollut Res 29, 15347–15363 (2022). https://doi.org/10.1007/s11356-021-18305-5 . Efforts can also include removal of non-native vegetation and/or replanting of native vegetation.
- For an in-depth look at salt marsh restoration efforts to date, refer to Adam 2019 “Salt Marsh Restoration”[2]Adam, Paul. “Salt Marsh Restoration.” Coastal Wetlands, 2019, pp. 817–861, https://doi.org/10.1016/b978-0-444-63893-9.00023-x.
CDR Potential
Estimated Sequestration Potential: 0.004 – 0.015 Gt CO2e/year[3]Hoegh-Guldberg, O., Northrop, E. et al. 2023. "The ocean as a solution to climate change: Updated opportunities for action." Special Report. Washington, DC: World Resources Institute. Available online at https://oceanpanel.org/publication/ocean-solutions-to-climate-change
Sequestration Durability: Decades -- > 1,000 years[4]Cross, J.N., Sweeney, C., Jewett, E.B., Feely, R.A., McElhany, P., Carter, B., Stein, T., Kitch, G.D., and Gledhill, D.K., 2023. Strategy for NOAA Carbon Dioxide Removal Research: A white paper documenting a potential NOAA CDR Science Strategy as an element of NOAA’s Climate Interventions Portfolio. NOAA Special Report. NOAA, Washington DC. DOI: 10.25923/gzke-8730 (Durability of sequestered carbon will depend on multiple variables and can vary greatly between individual ecosystems)
Challenges
- Little potential for expansion of current habitats due to extensive land conversion.
- Gaining access to suitable land for restoration projects may be difficult and expensive.
- Determining baseline historical extent for setting restoration objectives.
Key Knowledge Gaps
- Need accurate predictions of carbon sequestration which requires integrated modeling of ecological impacts, land-use, and climate effects (made more challenging by high variability in carbon burial rates[5]Williamson P and Gattuso J-P (2022) Carbon Removal Using Coastal Blue Carbon Ecosystems Is Uncertain and Unreliable, With Questionable Climatic Cost-Effectiveness. Front. Clim. 4:853666. doi: 10.3389/fclim.2022.853666 ).
- Discerning between competing feedbacks associated with climate and sea level rise, which can have both positive and negative impacts on ecosystems.
- Measurement of greenhouse gases other than CO2 (e.g., CH4 and N2O) that are produced and their significance to net sequestration.
- What is the fate of carbon produced outside of marshes and trapped in them?
- Total global areal existing extent remains poorly mapped, especially at high latitudes.
First Order Priorities
*Adapted from the Environmental Defense Fund report on Coastal Natural Climate Solutions[6]Collins, J. R., Boenish, R. E., Kleisner, K. M., Rader, D. N., Fujita, R. M., and Moritsch M. M. 2022. Coastal natural climate solutions: an assessment of scientific knowledge surrounding pathways for carbon dioxide removal and avoided emissions in nearshore blue carbon ecosystems. Environmental Defense Fund, New York, NY. www.edf.org/sites/default/files/2022-10/Coastal%20Natural%20Climate%20Solutions.pdf
- Conduct research to better understand and characterize the multiple carbon fluxes within salt marsh systems
- Improve predictions of carbon sequestered by salt marshes through modeling*
- Better knowledge of the relative contributions of carbon produced outside of (allochthonous) and within (autochthonous) marshes to salt marsh organic carbon pools, and the labilities of the carbon derived from these two sources*
- Investigate and characterize climate impacts, both positive and negative, on salt marsh systems*
- Sea level rise, warming, and increased coastal storm frequency and severity all need further investigation.
- Measure non-CO2 greenhouse gases that are produced and compare with sequestration capabilities to better understand the net sequestration of salt marshes*
- Better global mapping of the current extent of salt marshes, particularly at high latitudes, as well as habitat-suitability mapping/analysis*
Mechanism for CDR
- Most restoration efforts include recovery of tidal exchange (e.g., restoring hydrology and tidal flow), managed realignment, and sediment level amendment[1] . Efforts can also include removal of non-native vegetation and/or replanting of native vegetation.
- For an in-depth look at salt marsh restoration efforts to date, refer to Adam 2019 “Salt Marsh Restoration”[2]
CDR Potential
Estimated Sequestration Potential: 0.004 – 0.015 Gt CO2e/year[3]
Sequestration Durability: Decades -- > 1,000 years[4] (Durability of sequestered carbon will depend on multiple variables and can vary greatly between individual ecosystems)
Challenges
- Little potential for expansion of current habitats due to extensive land conversion.
- Gaining access to suitable land for restoration projects may be difficult and expensive.
- Determining baseline historical extent for setting restoration objectives.
Key Knowledge Gaps
- Need accurate predictions of carbon sequestration which requires integrated modeling of ecological impacts, land-use, and climate effects (made more challenging by high variability in carbon burial rates[5] ).
- Discerning between competing feedbacks associated with climate and sea level rise, which can have both positive and negative impacts on ecosystems.
- Measurement of greenhouse gases other than CO2 (e.g., CH4 and N2O) that are produced and their significance to net sequestration.
- What is the fate of carbon produced outside of marshes and trapped in them?
- Total global areal existing extent remains poorly mapped, especially at high latitudes.
First Order Priorities
*Adapted from the Environmental Defense Fund report on Coastal Natural Climate Solutions[6]
- Conduct research to better understand and characterize the multiple carbon fluxes within salt marsh systems
- Improve predictions of carbon sequestered by salt marshes through modeling*
- Better knowledge of the relative contributions of carbon produced outside of (allochthonous) and within (autochthonous) marshes to salt marsh organic carbon pools, and the labilities of the carbon derived from these two sources*
- Investigate and characterize climate impacts, both positive and negative, on salt marsh systems*
- Sea level rise, warming, and increased coastal storm frequency and severity all need further investigation.
- Measure non-CO2 greenhouse gases that are produced and compare with sequestration capabilities to better understand the net sequestration of salt marshes*
- Better global mapping of the current extent of salt marshes, particularly at high latitudes, as well as habitat-suitability mapping/analysis*
Seagrass Beds
Mechanisms for CDR
- Unlike salt marshes and mangrove forests, seagrass beds remove carbon from seawater, creating a disequilibrium in the CO2 concentration between the seawater and atmosphere. The resulting flux from the atmosphere into the seawater replenishes the deficit in dissolved inorganic carbon due to seagrass photosynthesis. This replenishment may be complete (which maximizes carbon removal) or incomplete (which results in an inefficiency in carbon removal accounting).
- In theory, restoration (e.g., seed/seedling dispersal or planting) is the most likely mechanism for CDR, however, efforts to date are often unsuccessful (Macreadie et al., 2021). Restoration propositions are further complicated by the outstanding questions around net carbon removal (especially under different climate change scenarios) and the extent of suitable locations.
- A 2023 study in Australia found that sediment carbon stocks show little recovery immediately after restoration and can take >5 years to return to expected carbon sequestration rates (Rahayu et al., 2023).
- Restoration of hydrology, through reversing drainage or removing tidal restrictions, may assist in the recovery and expansion of existing seagrass beds (NASEM, 2019). This path may be met with more resistance (especially political and social) than other types of restoration efforts.
CDR Potential
Estimated Sequestration Potential: 0.007 – 0.025 Gt CO2e/year (NASEM, 2019)
Sequestration Durability: Decades — 1,000 years (Cross et al., 2023) (Durability of sequestered carbon will depend on multiple variables and can vary greatly between individual ecosystems)
Challenges
- A 2023 review of all available literature on eelgrass (the dominant species of seagrass along the United States west coast) restoration projects in California, Oregon, and Washington found that of the 82 restoration projects, only 6 were published in peer-reviewed journals. This highlights the lack of data availability that can make assessing successes, failures, and best practices difficult (Ward & Beheshti, 2023).
- Difficult to differentiate between autochthonous and allochthonous sources of carbon in sediments (Macreadie et al., 2021)
- There is substantial inorganic carbon in seagrasses which is derived from outside the seagrass bed (Saderne et al., 2019) which in turn has sparked debate over whether seagrasses are ultimately a carbon sink or source.
- Seagrass restoration efforts are often unsuccessful (Macreadie et al., 2021)
- High variability in carbon burial rates (Williamson & Gattuso, 2022)
- High cost associated with restoration efforts (Vanderklift et al., 2018)
Key Knowledge Gaps
- Robust measurements of air-water CO2 exchange above seagrass beds
- Global extent of seagrasses (including future extent under changing climate)
- Causes of failed restoration projects
- What are the sources and fates of carbonates in seagrass beds?
First-Order Priorities
*Adapted from the Environmental Defense Fund report on Coastal Natural Climate Solutions (Collins et al., 2022)
- Take additional measurements of air-sea gas exchange above seagrass beds*
- This will lead to the development of more robust predictive models to better understand CDR efficacy.
- Map the global extent of seagrasses and their likely future extent*
- This is likely to involve some combination of active remote sensing and field surveys as well as habitat suitability analyses.
- Mapping of likely future extent will involve considerations of future climate change impacts.
- Continue investigations into the causes of restoration project failures in seagrass systems*
- This effort will likely involve better monitoring, recording, and publication of data on restoration efforts.
- Improve the understanding of the sources and fate of carbonates in seagrass bed sediments*
- This includes the contributions to CO2 production both within seagrass beds and upstream of these ecosystems.
Mechanisms for CDR
- Unlike salt marshes and mangrove forests, seagrass beds remove carbon from seawater, creating a disequilibrium in the CO2 concentration between the seawater and atmosphere. The resulting flux from the atmosphere into the seawater replenishes the deficit in dissolved inorganic carbon due to seagrass photosynthesis. This replenishment may be complete (which maximizes carbon removal) or incomplete (which results in an inefficiency in carbon removal accounting).
- In theory, restoration (e.g., seed/seedling dispersal or planting) is the most likely mechanism for CDR, however, efforts to date are often unsuccessful (Macreadie et al., 2021). Restoration propositions are further complicated by the outstanding questions around net carbon removal (especially under different climate change scenarios) and the extent of suitable locations.
- A 2023 study in Australia found that sediment carbon stocks show little recovery immediately after restoration and can take >5 years to return to expected carbon sequestration rates (Rahayu et al., 2023).
- Restoration of hydrology, through reversing drainage or removing tidal restrictions, may assist in the recovery and expansion of existing seagrass beds (NASEM, 2019). This path may be met with more resistance (especially political and social) than other types of restoration efforts.
CDR Potential
Estimated Sequestration Potential: 0.007 – 0.025 Gt CO2e/year (NASEM, 2019)
Sequestration Durability: Decades -- 1,000 years (Cross et al., 2023) (Durability of sequestered carbon will depend on multiple variables and can vary greatly between individual ecosystems)
Challenges
- A 2023 review of all available literature on eelgrass (the dominant species of seagrass along the United States west coast) restoration projects in California, Oregon, and Washington found that of the 82 restoration projects, only 6 were published in peer-reviewed journals. This highlights the lack of data availability that can make assessing successes, failures, and best practices difficult (Ward & Beheshti, 2023).
- Difficult to differentiate between autochthonous and allochthonous sources of carbon in sediments (Macreadie et al., 2021)
- There is substantial inorganic carbon in seagrasses which is derived from outside the seagrass bed (Saderne et al., 2019) which in turn has sparked debate over whether seagrasses are ultimately a carbon sink or source.
- Seagrass restoration efforts are often unsuccessful (Macreadie et al., 2021)
- High variability in carbon burial rates (Williamson & Gattuso, 2022)
- High cost associated with restoration efforts (Vanderklift et al., 2018)
Key Knowledge Gaps
- Robust measurements of air-water CO2 exchange above seagrass beds
- Global extent of seagrasses (including future extent under changing climate)
- Causes of failed restoration projects
- What are the sources and fates of carbonates in seagrass beds?
First-Order Priorities
*Adapted from the Environmental Defense Fund report on Coastal Natural Climate Solutions (Collins et al., 2022)
- Take additional measurements of air-sea gas exchange above seagrass beds*
- This will lead to the development of more robust predictive models to better understand CDR efficacy.
- Map the global extent of seagrasses and their likely future extent*
- This is likely to involve some combination of active remote sensing and field surveys as well as habitat suitability analyses.
- Mapping of likely future extent will involve considerations of future climate change impacts.
- Continue investigations into the causes of restoration project failures in seagrass systems*
- This effort will likely involve better monitoring, recording, and publication of data on restoration efforts.
- Improve the understanding of the sources and fate of carbonates in seagrass bed sediments*
- This includes the contributions to CO2 production both within seagrass beds and upstream of these ecosystems.
Mechanisms for CDR
- Unlike salt marshes and mangrove forests, seagrass beds remove carbon from seawater, creating a disequilibrium in the CO2 concentration between the seawater and atmosphere. The resulting flux from the atmosphere into the seawater replenishes the deficit in dissolved inorganic carbon due to seagrass photosynthesis. This replenishment may be complete (which maximizes carbon removal) or incomplete (which results in an inefficiency in carbon removal accounting).
- In theory, restoration (e.g., seed/seedling dispersal or planting) is the most likely mechanism for CDR, however, efforts to date are often unsuccessful (Macreadie et al., 2021). Restoration propositions are further complicated by the outstanding questions around net carbon removal (especially under different climate change scenarios) and the extent of suitable locations.
- A 2023 study in Australia found that sediment carbon stocks show little recovery immediately after restoration and can take >5 years to return to expected carbon sequestration rates (Rahayu et al., 2023).
- Restoration of hydrology, through reversing drainage or removing tidal restrictions, may assist in the recovery and expansion of existing seagrass beds[3]National Academies of Sciences, Engineering, and Medicine 2019. Negative Emissions Technologies and Reliable Sequestration: A Research Agenda. Washington, DC: The National Academies Press. https://doi.org/10.17226/25259. . This path may be met with more resistance (especially political and social) than other types of restoration efforts.
CDR Potential
Estimated Sequestration Potential: 0.007 – 0.025 Gt CO2e/year (NASEM, 2019)
Sequestration Durability: Decades -- 1,000 years (Cross et al., 2023) (Durability of sequestered carbon will depend on multiple variables and can vary greatly between individual ecosystems)
Challenges
- A 2023 review of all available literature on eelgrass (the dominant species of seagrass along the United States west coast) restoration projects in California, Oregon, and Washington found that of the 82 restoration projects, only 6 were published in peer-reviewed journals. This highlights the lack of data availability that can make assessing successes, failures, and best practices difficult (Ward & Beheshti, 2023).
- Difficult to differentiate between autochthonous and allochthonous sources of carbon in sediments (Macreadie et al., 2021)
- There is substantial inorganic carbon in seagrasses which is derived from outside the seagrass bed (Saderne et al., 2019) which in turn has sparked debate over whether seagrasses are ultimately a carbon sink or source.
- Seagrass restoration efforts are often unsuccessful (Macreadie et al., 2021)
- High variability in carbon burial rates (Williamson & Gattuso, 2022)
- High cost associated with restoration efforts (Vanderklift et al., 2018)
Key Knowledge Gaps
- Robust measurements of air-water CO2 exchange above seagrass beds
- Global extent of seagrasses (including future extent under changing climate)
- Causes of failed restoration projects
- What are the sources and fates of carbonates in seagrass beds?
First-Order Priorities
*Adapted from the Environmental Defense Fund report on Coastal Natural Climate Solutions (Collins et al., 2022)
- Take additional measurements of air-sea gas exchange above seagrass beds*
- This will lead to the development of more robust predictive models to better understand CDR efficacy.
- Map the global extent of seagrasses and their likely future extent*
- This is likely to involve some combination of active remote sensing and field surveys as well as habitat suitability analyses.
- Mapping of likely future extent will involve considerations of future climate change impacts.
- Continue investigations into the causes of restoration project failures in seagrass systems*
- This effort will likely involve better monitoring, recording, and publication of data on restoration efforts.
- Improve the understanding of the sources and fate of carbonates in seagrass bed sediments*
- This includes the contributions to CO2 production both within seagrass beds and upstream of these ecosystems.
Mechanisms for CDR
- Unlike salt marshes and mangrove forests, seagrass beds remove carbon from seawater, creating a disequilibrium in the CO2 concentration between the seawater and atmosphere. The resulting flux from the atmosphere into the seawater replenishes the deficit in dissolved inorganic carbon due to seagrass photosynthesis. This replenishment may be complete (which maximizes carbon removal) or incomplete (which results in an inefficiency in carbon removal accounting).
- In theory, restoration (e.g., seed/seedling dispersal or planting) is the most likely mechanism for CDR, however, efforts to date are often unsuccessful. Restoration propositions are further complicated by the outstanding questions around net carbon removal (especially under different climate change scenarios) and the extent of suitable locations.
- A 2023 study in Australia found that sediment carbon stocks show little recovery immediately after restoration and can take >5 years to return to expected carbon sequestration rates.
- Restoration of hydrology, through reversing drainage or removing tidal restrictions, may assist in the recovery and expansion of existing seagrass beds. This path may be met with more resistance (especially political and social) than other types of restoration efforts.
CDR Potential
Estimated Sequestration Potential: 0.007 – 0.025 Gt CO2e/year
Sequestration Durability: Decades -- 1,000 years (Durability of sequestered carbon will depend on multiple variables and can vary greatly between individual ecosystems)
Challenges
- A 2023 review of all available literature on eelgrass (the dominant species of seagrass along the United States west coast) restoration projects in California, Oregon, and Washington found that of the 82 restoration projects, only 6 were published in peer-reviewed journals. This highlights the lack of data availability that can make assessing successes, failures, and best practices difficult.
- Difficult to differentiate between autochthonous and allochthonous sources of carbon in sediments
- There is substantial inorganic carbon in seagrasses which is derived from outside the seagrass bed which in turn has sparked debate over whether seagrasses are ultimately a carbon sink or source.
- Seagrass restoration efforts are often unsuccessful
- High variability in carbon burial rates
- High cost associated with restoration efforts
Key Knowledge Gaps
- Robust measurements of air-water CO2 exchange above seagrass beds
- Global extent of seagrasses (including future extent under changing climate)
- Causes of failed restoration projects
- What are the sources and fates of carbonates in seagrass beds?
First-Order Priorities
*Adapted from the Environmental Defense Fund report on Coastal Natural Climate Solutions
- Take additional measurements of air-sea gas exchange above seagrass beds*
- This will lead to the development of more robust predictive models to better understand CDR efficacy.
- Map the global extent of seagrasses and their likely future extent*
- This is likely to involve some combination of active remote sensing and field surveys as well as habitat suitability analyses.
- Mapping of likely future extent will involve considerations of future climate change impacts.
- Continue investigations into the causes of restoration project failures in seagrass systems*
- This effort will likely involve better monitoring, recording, and publication of data on restoration efforts.
- Improve the understanding of the sources and fate of carbonates in seagrass bed sediments*
- This includes the contributions to CO2 production both within seagrass beds and upstream of these ecosystems.
Mechanisms for CDR
- Unlike salt marshes and mangrove forests, seagrass beds remove carbon from seawater, creating a disequilibrium in the CO2 concentration between the seawater and atmosphere. The resulting flux from the atmosphere into the seawater replenishes the deficit in dissolved inorganic carbon due to seagrass photosynthesis. This replenishment may be complete (which maximizes carbon removal) or incomplete (which results in an inefficiency in carbon removal accounting).
- In theory, restoration (e.g., seed/seedling dispersal or planting) is the most likely mechanism for CDR, however, efforts to date are often unsuccessful[1]Macreadie, P.I., Costa, M.D.P., Atwood, T.B. et al. Blue carbon as a natural climate solution. Nat Rev Earth Environ 2, 826–839 (2021). https://doi.org/10.1038/s43017-021-00224-1
. Restoration propositions are further complicated by the outstanding questions around net carbon removal (especially under different climate change scenarios) and the extent of suitable locations.
- A 2023 study in Australia found that sediment carbon stocks show little recovery immediately after restoration and can take >5 years to return to expected carbon sequestration rates[2]Rahayu, Yusmiana P., et al. “Little change in surface sediment carbon stock following seagrass restoration in Shark Bay, Western Australia.” Estuarine, Coastal and Shelf Science, vol. 294, 2023, p. 108535, https://doi.org/10.1016/j.ecss.2023.108535. .
- Restoration of hydrology, through reversing drainage or removing tidal restrictions, may assist in the recovery and expansion of existing seagrass beds[3]National Academies of Sciences, Engineering, and Medicine 2019. Negative Emissions Technologies and Reliable Sequestration: A Research Agenda. Washington, DC: The National Academies Press. https://doi.org/10.17226/25259. . This path may be met with more resistance (especially political and social) than other types of restoration efforts.
CDR Potential
Estimated Sequestration Potential: 0.007 – 0.025 Gt CO2e/year[4]Hoegh-Guldberg, O., Northrop, E. et al. 2023. "The ocean as a solution to climate change: Updated opportunities for action." Special Report. Washington, DC: World Resources Institute. Available online at https://oceanpanel.org/publication/ocean-solutions-to-climate-change
Sequestration Durability: Decades -- 1,000 years[5]Cross, J.N., Sweeney, C., Jewett, E.B., Feely, R.A., McElhany, P., Carter, B., Stein, T., Kitch, G.D., and Gledhill, D.K., 2023. Strategy for NOAA Carbon Dioxide Removal Research: A white paper documenting a potential NOAA CDR Science Strategy as an element of NOAA’s Climate Interventions Portfolio. NOAA Special Report. NOAA, Washington DC. DOI: 10.25923/gzke-8730 (Durability of sequestered carbon will depend on multiple variables and can vary greatly between individual ecosystems)
Challenges
- A 2023 review of all available literature on eelgrass (the dominant species of seagrass along the United States west coast) restoration projects in California, Oregon, and Washington found that of the 82 restoration projects, only 6 were published in peer-reviewed journals. This highlights the lack of data availability that can make assessing successes, failures, and best practices difficult[6]Ward, Melissa, and Kathryn Beheshti. “Lessons learned from over thirty years of eelgrass restoration on the US West Coast.” Ecosphere, vol. 14, no. 8, 2023, https://doi.org/10.1002/ecs2.4642. .
- Difficult to differentiate between autochthonous and allochthonous sources of carbon in sediments[7]Macreadie, P.I., Costa, M.D.P., Atwood, T.B. et al. Blue carbon as a natural climate solution. Nat Rev Earth Environ 2, 826–839 (2021). https://doi.org/10.1038/s43017-021-00224-1
- There is substantial inorganic carbon in seagrasses which is derived from outside the seagrass bed[8]Saderne, V., Geraldi, N.R., Macreadie, P.I. et al. Role of carbonate burial in Blue Carbon budgets. Nat Commun 10, 1106 (2019). https://doi.org/10.1038/s41467-019-08842-6 which in turn has sparked debate over whether seagrasses are ultimately a carbon sink or source.
- Seagrass restoration efforts are often unsuccessful[9]Macreadie, P.I., Costa, M.D.P., Atwood, T.B. et al. Blue carbon as a natural climate solution. Nat Rev Earth Environ 2, 826–839 (2021). https://doi.org/10.1038/s43017-021-00224-1
- High variability in carbon burial rates[10]Williamson P and Gattuso J-P (2022) Carbon Removal Using Coastal Blue Carbon Ecosystems Is Uncertain and Unreliable, With Questionable Climatic Cost-Effectiveness. Front. Clim. 4:853666. doi: 10.3389/fclim.2022.853666
- High cost associated with restoration efforts[11]Vanderklift M, Steven A, Marcos-Martinez R, Gorman D (2018) Achieving carbon offsets through blue carbon: a review of needs and opportunities relevant to the Australian seafood industry, FRDC Final Report 2018/060. CSIRO Oceans and Atmosphere, Crawley, November 2018.
Key Knowledge Gaps
- Robust measurements of air-water CO2 exchange above seagrass beds
- Global extent of seagrasses (including future extent under changing climate)
- Causes of failed restoration projects
- What are the sources and fates of carbonates in seagrass beds?
First-Order Priorities
*Adapted from the Environmental Defense Fund report on Coastal Natural Climate Solutions[12]Collins, J. R., Boenish, R. E., Kleisner, K. M., Rader, D. N., Fujita, R. M., and Moritsch M. M. 2022. Coastal natural climate solutions: an assessment of scientific knowledge surrounding pathways for carbon dioxide removal and avoided emissions in nearshore blue carbon ecosystems. Environmental Defense Fund, New York, NY. https://www.edf.org/sites/default/files/2022-10/Coastal%20Natural%20Climate%20Solutions.pdf
- Take additional measurements of air-sea gas exchange above seagrass beds*
- This will lead to the development of more robust predictive models to better understand CDR efficacy.
- Map the global extent of seagrasses and their likely future extent*
- This is likely to involve some combination of active remote sensing and field surveys as well as habitat suitability analyses.
- Mapping of likely future extent will involve considerations of future climate change impacts.
- Continue investigations into the causes of restoration project failures in seagrass systems*
- This effort will likely involve better monitoring, recording, and publication of data on restoration efforts.
- Improve the understanding of the sources and fate of carbonates in seagrass bed sediments*
- This includes the contributions to CO2 production both within seagrass beds and upstream of these ecosystems.
Mechanisms for CDR
- Unlike salt marshes and mangrove forests, seagrass beds remove carbon from seawater, creating a disequilibrium in the CO2 concentration between the seawater and atmosphere. The resulting flux from the atmosphere into the seawater replenishes the deficit in dissolved inorganic carbon due to seagrass photosynthesis. This replenishment may be complete (which maximizes carbon removal) or incomplete (which results in an inefficiency in carbon removal accounting).
- In theory, restoration (e.g., seed/seedling dispersal or planting) is the most likely mechanism for CDR, however, efforts to date are often unsuccessful[1]Macreadie, P.I., Costa, M.D.P., Atwood, T.B. et al. Blue carbon as a natural climate solution. Nat Rev Earth Environ 2, 826–839 (2021). https://doi.org/10.1038/s43017-021-00224-1
. Restoration propositions are further complicated by the outstanding questions around net carbon removal (especially under different climate change scenarios) and the extent of suitable locations.
- A 2023 study in Australia found that sediment carbon stocks show little recovery immediately after restoration and can take >5 years to return to expected carbon sequestration rates[2]Rahayu, Yusmiana P., et al. “Little change in surface sediment carbon stock following seagrass restoration in Shark Bay, Western Australia.” Estuarine, Coastal and Shelf Science, vol. 294, 2023, p. 108535, https://doi.org/10.1016/j.ecss.2023.108535. .
- Restoration of hydrology, through reversing drainage or removing tidal restrictions, may assist in the recovery and expansion of existing seagrass beds[3]National Academies of Sciences, Engineering, and Medicine 2019. Negative Emissions Technologies and Reliable Sequestration: A Research Agenda. Washington, DC: The National Academies Press. https://doi.org/10.17226/25259. . This path may be met with more resistance (especially political and social) than other types of restoration efforts.
CDR Potential
Estimated Sequestration Potential: 0.007 – 0.025 Gt CO2e/year[4]Hoegh-Guldberg, O., Northrop, E. et al. 2023. "The ocean as a solution to climate change: Updated opportunities for action." Special Report. Washington, DC: World Resources Institute. Available online at https://oceanpanel.org/publication/ocean-solutions-to-climate-change
Sequestration Durability: Decades -- 1,000 years[5]Cross, J.N., Sweeney, C., Jewett, E.B., Feely, R.A., McElhany, P., Carter, B., Stein, T., Kitch, G.D., and Gledhill, D.K., 2023. Strategy for NOAA Carbon Dioxide Removal Research: A white paper documenting a potential NOAA CDR Science Strategy as an element of NOAA’s Climate Interventions Portfolio. NOAA Special Report. NOAA, Washington DC. DOI: 10.25923/gzke-8730 (Durability of sequestered carbon will depend on multiple variables and can vary greatly between individual ecosystems)
Challenges
- A 2023 review of all available literature on eelgrass (the dominant species of seagrass along the United States west coast) restoration projects in California, Oregon, and Washington found that of the 82 restoration projects, only 6 were published in peer-reviewed journals. This highlights the lack of data availability that can make assessing successes, failures, and best practices difficult[6]Ward, Melissa, and Kathryn Beheshti. “Lessons learned from over thirty years of eelgrass restoration on the US West Coast.” Ecosphere, vol. 14, no. 8, 2023, https://doi.org/10.1002/ecs2.4642. .
- Difficult to differentiate between autochthonous and allochthonous sources of carbon in sediments[7]Macreadie, P.I., Costa, M.D.P., Atwood, T.B. et al. Blue carbon as a natural climate solution. Nat Rev Earth Environ 2, 826–839 (2021). https://doi.org/10.1038/s43017-021-00224-1
- There is substantial inorganic carbon in seagrasses which is derived from outside the seagrass bed[8]Saderne, V., Geraldi, N.R., Macreadie, P.I. et al. Role of carbonate burial in Blue Carbon budgets. Nat Commun 10, 1106 (2019). https://doi.org/10.1038/s41467-019-08842-6 which in turn has sparked debate over whether seagrasses are ultimately a carbon sink or source.
- Seagrass restoration efforts are often unsuccessful[9]Macreadie, P.I., Costa, M.D.P., Atwood, T.B. et al. Blue carbon as a natural climate solution. Nat Rev Earth Environ 2, 826–839 (2021). https://doi.org/10.1038/s43017-021-00224-1
- High variability in carbon burial rates[10]Williamson P and Gattuso J-P (2022) Carbon Removal Using Coastal Blue Carbon Ecosystems Is Uncertain and Unreliable, With Questionable Climatic Cost-Effectiveness. Front. Clim. 4:853666. doi: 10.3389/fclim.2022.853666
- High cost associated with restoration efforts[11]Vanderklift M, Steven A, Marcos-Martinez R, Gorman D (2018) Achieving carbon offsets through blue carbon: a review of needs and opportunities relevant to the Australian seafood industry, FRDC Final Report 2018/060. CSIRO Oceans and Atmosphere, Crawley, November 2018.
Key Knowledge Gaps
- Robust measurements of air-water CO2 exchange above seagrass beds
- Global extent of seagrasses (including future extent under changing climate)
- Causes of failed restoration projects
- What are the sources and fates of carbonates in seagrass beds?
First Order Priorities
*Adapted from the Environmental Defense Fund report on Coastal Natural Climate Solutions[12]Collins, J. R., Boenish, R. E., Kleisner, K. M., Rader, D. N., Fujita, R. M., and Moritsch M. M. 2022. Coastal natural climate solutions: an assessment of scientific knowledge surrounding pathways for carbon dioxide removal and avoided emissions in nearshore blue carbon ecosystems. Environmental Defense Fund, New York, NY. www.edf.org/sites/default/files/2022-10/Coastal%20Natural%20Climate%20Solutions.pdf
- Take additional measurements of air-sea gas exchange above seagrass beds*
- This will lead to the development of more robust predictive models to better understand CDR efficacy.
- Map the global extent of seagrasses and their likely future extent*
- This is likely to involve some combination of active remote sensing and field surveys as well as habitat suitability analyses.
- Mapping of likely future extent will involve considerations of future climate change impacts.
- Continue investigations into the causes of restoration project failures in seagrass systems*
- This effort will likely involve better monitoring, recording, and publication of data on restoration efforts.
- Improve the understanding of the sources and fate of carbonates in seagrass bed sediments*
- This includes the contributions to CO2 production both within seagrass beds and upstream of these ecosystems.
Mechanisms for CDR
- Unlike salt marshes and mangrove forests, seagrass beds remove carbon from seawater, creating a disequilibrium in the CO2 concentration between the seawater and atmosphere. The resulting flux from the atmosphere into the seawater replenishes the deficit in dissolved inorganic carbon due to seagrass photosynthesis. This replenishment may be complete (which maximizes carbon removal) or incomplete (which results in an inefficiency in carbon removal accounting).
- In theory, restoration (e.g., seed/seedling dispersal or planting) is the most likely mechanism for CDR, however, efforts to date are often unsuccessful[1]Macreadie, P.I., Costa, M.D.P., Atwood, T.B. et al. Blue carbon as a natural climate solution. Nat Rev Earth Environ 2, 826–839 (2021). https://doi.org/10.1038/s43017-021-00224-1
. Restoration propositions are further complicated by the outstanding questions around net carbon removal (especially under different climate change scenarios) and the extent of suitable locations.
- A 2023 study in Australia found that sediment carbon stocks show little recovery immediately after restoration and can take >5 years to return to expected carbon sequestration rates[2]Rahayu, Yusmiana P., et al. “Little change in surface sediment carbon stock following seagrass restoration in Shark Bay, Western Australia.” Estuarine, Coastal and Shelf Science, vol. 294, 2023, p. 108535, https://doi.org/10.1016/j.ecss.2023.108535. .
- Restoration of hydrology, through reversing drainage or removing tidal restrictions, may assist in the recovery and expansion of existing seagrass beds[3]National Academies of Sciences, Engineering, and Medicine 2019. Negative Emissions Technologies and Reliable Sequestration: A Research Agenda. Washington, DC: The National Academies Press. https://doi.org/10.17226/25259. . This path may be met with more resistance (especially political and social) than other types of restoration efforts.
CDR Potential
Estimated Sequestration Potential: 0.007 – 0.025 Gt CO2e/year[4]Hoegh-Guldberg, O., Northrop, E. et al. 2023. "The ocean as a solution to climate change: Updated opportunities for action." Special Report. Washington, DC: World Resources Institute. Available online at https://oceanpanel.org/publication/ocean-solutions-to-climate-change
Sequestration Durability: Decades -- 1,000 years[5]Cross, J.N., Sweeney, C., Jewett, E.B., Feely, R.A., McElhany, P., Carter, B., Stein, T., Kitch, G.D., and Gledhill, D.K., 2023. Strategy for NOAA Carbon Dioxide Removal Research: A white paper documenting a potential NOAA CDR Science Strategy as an element of NOAA’s Climate Interventions Portfolio. NOAA Special Report. NOAA, Washington DC. DOI: 10.25923/gzke-8730 (Durability of sequestered carbon will depend on multiple variables and can vary greatly between individual ecosystems)
Challenges
- A 2023 review of all available literature on eelgrass (the dominant species of seagrass along the United States west coast) restoration projects in California, Oregon, and Washington found that of the 82 restoration projects, only 6 were published in peer-reviewed journals. This highlights the lack of data availability that can make assessing successes, failures, and best practices difficult[6]Ward, Melissa, and Kathryn Beheshti. “Lessons learned from over thirty years of eelgrass restoration on the US West Coast.” Ecosphere, vol. 14, no. 8, 2023, https://doi.org/10.1002/ecs2.4642. .
- Difficult to differentiate between autochthonous and allochthonous sources of carbon in sediments[7]Macreadie, P.I., Costa, M.D.P., Atwood, T.B. et al. Blue carbon as a natural climate solution. Nat Rev Earth Environ 2, 826–839 (2021). https://doi.org/10.1038/s43017-021-00224-1
- There is substantial inorganic carbon in seagrasses which is derived from outside the seagrass bed[8]Saderne, V., Geraldi, N.R., Macreadie, P.I. et al. Role of carbonate burial in Blue Carbon budgets. Nat Commun 10, 1106 (2019). https://doi.org/10.1038/s41467-019-08842-6 which in turn has sparked debate over whether seagrasses are ultimately a carbon sink or source.
- Seagrass restoration efforts are often unsuccessful[9]Macreadie, P.I., Costa, M.D.P., Atwood, T.B. et al. Blue carbon as a natural climate solution. Nat Rev Earth Environ 2, 826–839 (2021). https://doi.org/10.1038/s43017-021-00224-1
- High variability in carbon burial rates[10]Williamson P and Gattuso J-P (2022) Carbon Removal Using Coastal Blue Carbon Ecosystems Is Uncertain and Unreliable, With Questionable Climatic Cost-Effectiveness. Front. Clim. 4:853666. doi: 10.3389/fclim.2022.853666
- High cost associated with restoration efforts[11]Vanderklift M, Steven A, Marcos-Martinez R, Gorman D (2018) Achieving carbon offsets through blue carbon: a review of needs and opportunities relevant to the Australian seafood industry, FRDC Final Report 2018/060. CSIRO Oceans and Atmosphere, Crawley, November 2018.
Key Knowledge Gaps
- Robust measurements of air-water CO2 exchange above seagrass beds
- Global extent of seagrasses (including future extent under changing climate)
- Causes of failed restoration projects
- What are the sources and fates of carbonates in seagrass beds?
First Order Priorities
*Adapted from the Environmental Defense Fund report on Coastal Natural Climate Solutions[12]Collins, J. R., Boenish, R. E., Kleisner, K. M., Rader, D. N., Fujita, R. M., and Moritsch M. M. 2022. Coastal natural climate solutions: an assessment of scientific knowledge surrounding pathways for carbon dioxide removal and avoided emissions in nearshore blue carbon ecosystems. Environmental Defense Fund, New York, NY. www.edf.org/sites/default/files/2022-10/Coastal%20Natural%20Climate%20Solutions.pdf
- Take additional measurements of air-sea gas exchange above seagrass beds*
- This will lead to the development of more robust predictive models to better understand CDR efficacy.
- Map the global extent of seagrasses and their likely future extent*
- This is likely to involve some combination of active remote sensing and field surveys as well as habitat suitability analyses.
- Mapping of likely future extent will involve considerations of future climate change impacts.
- Continue investigations into the causes of restoration project failures in seagrass systems*
- This effort will likely involve better monitoring, recording, and publication of data on restoration efforts.
- Improve the understanding of the sources and fate of carbonates in seagrass bed sediments*
- This includes the contributions to CO2 production both within seagrass beds and upstream of these ecosystems.
Mechanisms for CDR
- Unlike salt marshes and mangrove forests, seagrass beds remove carbon from seawater, creating a disequilibrium in the CO2 concentration between the seawater and atmosphere. The resulting flux from the atmosphere into the seawater replenishes the deficit in dissolved inorganic carbon due to seagrass photosynthesis. This replenishment may be complete (which maximizes carbon removal) or incomplete (which results in an inefficiency in carbon removal accounting).
- In theory, restoration (e.g., seed/seedling dispersal or planting) is the most likely mechanism for CDR, however, efforts to date are often unsuccessful[1]Macreadie, P.I., Costa, M.D.P., Atwood, T.B. et al. Blue carbon as a natural climate solution. Nat Rev Earth Environ 2, 826–839 (2021). https://doi.org/10.1038/s43017-021-00224-1
. Restoration propositions are further complicated by the outstanding questions around net carbon removal (especially under different climate change scenarios) and the extent of suitable locations.
- A 2023 study in Australia found that sediment carbon stocks show little recovery immediately after restoration and can take >5 years to return to expected carbon sequestration rates[2]Rahayu, Yusmiana P., et al. “Little change in surface sediment carbon stock following seagrass restoration in Shark Bay, Western Australia.” Estuarine, Coastal and Shelf Science, vol. 294, 2023, p. 108535, https://doi.org/10.1016/j.ecss.2023.108535. .
- Restoration of hydrology, through reversing drainage or removing tidal restrictions, may assist in the recovery and expansion of existing seagrass beds[3]National Academies of Sciences, Engineering, and Medicine 2019. Negative Emissions Technologies and Reliable Sequestration: A Research Agenda. Washington, DC: The National Academies Press. https://doi.org/10.17226/25259. . This path may be met with more resistance (especially political and social) than other types of restoration efforts.
CDR Potential
Estimated Sequestration Potential: 0.007 – 0.025 Gt CO2e/year[4]Hoegh-Guldberg, O., Northrop, E. et al. 2023. "The ocean as a solution to climate change: Updated opportunities for action." Special Report. Washington, DC: World Resources Institute. Available online at https://oceanpanel.org/publication/ocean-solutions-to-climate-change
Sequestration Durability: Decades -- > 1,000 years[5]Cross, J.N., Sweeney, C., Jewett, E.B., Feely, R.A., McElhany, P., Carter, B., Stein, T., Kitch, G.D., and Gledhill, D.K., 2023. Strategy for NOAA Carbon Dioxide Removal Research: A white paper documenting a potential NOAA CDR Science Strategy as an element of NOAA’s Climate Interventions Portfolio. NOAA Special Report. NOAA, Washington DC. DOI: 10.25923/gzke-8730 (Durability of sequestered carbon will depend on multiple variables and can vary greatly between individual ecosystems)
Challenges
- A 2023 review of all available literature on eelgrass (the dominant species of seagrass along the United States west coast) restoration projects in California, Oregon, and Washington found that of the 82 restoration projects, only 6 were published in peer-reviewed journals. This highlights the lack of data availability that can make assessing successes, failures, and best practices difficult[6]Ward, Melissa, and Kathryn Beheshti. “Lessons learned from over thirty years of eelgrass restoration on the US West Coast.” Ecosphere, vol. 14, no. 8, 2023, https://doi.org/10.1002/ecs2.4642. .
- Difficult to differentiate between autochthonous and allochthonous sources of carbon in sediments[7]Macreadie, P.I., Costa, M.D.P., Atwood, T.B. et al. Blue carbon as a natural climate solution. Nat Rev Earth Environ 2, 826–839 (2021). https://doi.org/10.1038/s43017-021-00224-1
- There is substantial inorganic carbon in seagrasses which is derived from outside the seagrass bed[8]Saderne, V., Geraldi, N.R., Macreadie, P.I. et al. Role of carbonate burial in Blue Carbon budgets. Nat Commun 10, 1106 (2019). https://doi.org/10.1038/s41467-019-08842-6 which in turn has sparked debate over whether seagrasses are ultimately a carbon sink or source.
- Seagrass restoration efforts are often unsuccessful[9]Macreadie, P.I., Costa, M.D.P., Atwood, T.B. et al. Blue carbon as a natural climate solution. Nat Rev Earth Environ 2, 826–839 (2021). https://doi.org/10.1038/s43017-021-00224-1
- High variability in carbon burial rates[10]Williamson P and Gattuso J-P (2022) Carbon Removal Using Coastal Blue Carbon Ecosystems Is Uncertain and Unreliable, With Questionable Climatic Cost-Effectiveness. Front. Clim. 4:853666. doi: 10.3389/fclim.2022.853666
- High cost associated with restoration efforts[11]Vanderklift M, Steven A, Marcos-Martinez R, Gorman D (2018) Achieving carbon offsets through blue carbon: a review of needs and opportunities relevant to the Australian seafood industry, FRDC Final Report 2018/060. CSIRO Oceans and Atmosphere, Crawley, November 2018.
Key Knowledge Gaps
- Robust measurements of air-water CO2 exchange above seagrass beds
- Global extent of seagrasses (including future extent under changing climate)
- Causes of failed restoration projects
- What are the sources and fates of carbonates in seagrass beds?
First Order Priorities
*Adapted from the Environmental Defense Fund report on Coastal Natural Climate Solutions[12]Collins, J. R., Boenish, R. E., Kleisner, K. M., Rader, D. N., Fujita, R. M., and Moritsch M. M. 2022. Coastal natural climate solutions: an assessment of scientific knowledge surrounding pathways for carbon dioxide removal and avoided emissions in nearshore blue carbon ecosystems. Environmental Defense Fund, New York, NY. www.edf.org/sites/default/files/2022-10/Coastal%20Natural%20Climate%20Solutions.pdf
- Take additional measurements of air-sea gas exchange above seagrass beds*
- This will lead to the development of more robust predictive models to better understand CDR efficacy.
- Map the global extent of seagrasses and their likely future extent*
- This is likely to involve some combination of active remote sensing and field surveys as well as habitat suitability analyses.
- Mapping of likely future extent will involve considerations of future climate change impacts.
- Continue investigations into the causes of restoration project failures in seagrass systems*
- This effort will likely involve better monitoring, recording, and publication of data on restoration efforts.
- Improve the understanding of the sources and fate of carbonates in seagrass bed sediments*
- This includes the contributions to CO2 production both within seagrass beds and upstream of these ecosystems.
Mechanisms for CDR
- Unlike salt marshes and mangrove forests, seagrass beds remove carbon from seawater, creating a disequilibrium in the CO2 concentration between the seawater and atmosphere. The resulting flux from the atmosphere into the seawater replenishes the deficit in dissolved inorganic carbon due to seagrass photosynthesis. This replenishment may be complete (which maximizes carbon removal) or incomplete (which results in an inefficiency in carbon removal accounting).
- In theory, restoration (e.g., seed/seedling dispersal or planting) is the most likely mechanism for CDR, however, efforts to date are often unsuccessful[1]Macreadie, P.I., Costa, M.D.P., Atwood, T.B. et al. Blue carbon as a natural climate solution. Nat Rev Earth Environ 2, 826–839 (2021). https://doi.org/10.1038/s43017-021-00224-1
. Restoration propositions are further complicated by the outstanding questions around net carbon removal (especially under different climate change scenarios) and the extent of suitable locations.
- A 2023 study in Australia found that sediment carbon stocks show little recovery immediately after restoration and can take >5 years to return to expected carbon sequestration rates[2]Rahayu, Yusmiana P., et al. “Little change in surface sediment carbon stock following seagrass restoration in Shark Bay, Western Australia.” Estuarine, Coastal and Shelf Science, vol. 294, 2023, p. 108535, https://doi.org/10.1016/j.ecss.2023.108535. .
- Restoration of hydrology, through reversing drainage or removing tidal restrictions, may assist in the recovery and expansion of existing seagrass beds[3]National Academies of Sciences, Engineering, and Medicine 2019. Negative Emissions Technologies and Reliable Sequestration: A Research Agenda. Washington, DC: The National Academies Press. https://doi.org/10.17226/25259. . This path may be met with more resistance (especially political and social) than other types of restoration efforts.
CDR Potential
Estimated Sequestration Potential: 0.007 – 0.025 Gt CO2e/year[4]Hoegh-Guldberg, O., Northrop, E. et al. 2023. "The ocean as a solution to climate change: Updated opportunities for action." Special Report. Washington, DC: World Resources Institute. Available online at https://oceanpanel.org/publication/ocean-solutions-to-climate-change
Sequestration Durability: Decades -- > 1,000 years[5]Cross, J.N., Sweeney, C., Jewett, E.B., Feely, R.A., McElhany, P., Carter, B., Stein, T., Kitch, G.D., and Gledhill, D.K., 2023. Strategy for NOAA Carbon Dioxide Removal Research: A white paper documenting a potential NOAA CDR Science Strategy as an element of NOAA’s Climate Interventions Portfolio. NOAA Special Report. NOAA, Washington DC. DOI: 10.25923/gzke-8730 (Durability of sequestered carbon will depend on multiple variables and can vary greatly between individual ecosystems)
Challenges
- A 2023 review of all available literature on eelgrass (the dominant species of seagrass along the United States west coast) restoration projects in California, Oregon, and Washington found that of the 82 restoration projects, only 6 were published in peer-reviewed journals. This highlights the lack of data availability that can make assessing successes, failures, and best practices difficult[6]Ward, Melissa, and Kathryn Beheshti. “Lessons learned from over thirty years of eelgrass restoration on the US West Coast.” Ecosphere, vol. 14, no. 8, 2023, https://doi.org/10.1002/ecs2.4642. .
- Difficult to differentiate between autochthonous and allochthonous sources of carbon in sediments[7]Macreadie, P.I., Costa, M.D.P., Atwood, T.B. et al. Blue carbon as a natural climate solution. Nat Rev Earth Environ 2, 826–839 (2021). https://doi.org/10.1038/s43017-021-00224-1
- There is substantial inorganic carbon in seagrasses which is derived from outside the seagrass bed[8]Saderne, V., Geraldi, N.R., Macreadie, P.I. et al. Role of carbonate burial in Blue Carbon budgets. Nat Commun 10, 1106 (2019). https://doi.org/10.1038/s41467-019-08842-6 which in turn has sparked debate over whether seagrasses are ultimately a carbon sink or source.
- Seagrass restoration efforts are often unsuccessful[9]Macreadie, P.I., Costa, M.D.P., Atwood, T.B. et al. Blue carbon as a natural climate solution. Nat Rev Earth Environ 2, 826–839 (2021). https://doi.org/10.1038/s43017-021-00224-1
- High variability in carbon burial rates[10]Williamson P and Gattuso J-P (2022) Carbon Removal Using Coastal Blue Carbon Ecosystems Is Uncertain and Unreliable, With Questionable Climatic Cost-Effectiveness. Front. Clim. 4:853666. doi: 10.3389/fclim.2022.853666
- High cost associated with restoration efforts[11]Vanderklift M, Steven A, Marcos-Martinez R, Gorman D (2018) Achieving carbon offsets through blue carbon: a review of needs and opportunities relevant to the Australian seafood industry, FRDC Final Report 2018/060. CSIRO Oceans and Atmosphere, Crawley, November 2018.
Key Knowledge Gaps
- Robust measurements of air-water CO2 exchange above seagrass beds
- Global extent of seagrasses (including future extent under changing climate)
- Causes of failed restoration projects
- What are the sources and fates of carbonates in seagrass beds?
First Order Priorities
*Adapted from the Environmental Defense Fund report on Coastal Natural Climate Solutions[12]Collins, J. R., Boenish, R. E., Kleisner, K. M., Rader, D. N., Fujita, R. M., and Moritsch M. M. 2022. Coastal natural climate solutions: an assessment of scientific knowledge surrounding pathways for carbon dioxide removal and avoided emissions in nearshore blue carbon ecosystems. Environmental Defense Fund, New York, NY. www.edf.org/sites/default/files/2022-10/Coastal%20Natural%20Climate%20Solutions.pdf
- Take additional measurements of air-sea gas exchange above seagrass beds*
- This will lead to the development of more robust predictive models to better understand CDR efficacy.
- Map the global extent of seagrasses and their likely future extent*
- This is likely to involve some combination of active remote sensing and field surveys as well as habitat suitability analyses.
- Mapping of likely future extent will involve considerations of future climate change impacts.
- Continue investigations into the causes of restoration project failures in seagrass systems*
- This effort will likely involve better monitoring, recording, and publication of data on restoration efforts.
- Improve the understanding of the sources and fate of carbonates in seagrass bed sediments*
- This includes the contributions to CO2 production both within seagrass beds and upstream of these ecosystems.
Mechanisms for CDR
- Unlike salt marshes and mangrove forests, seagrass beds remove carbon from seawater, creating a disequilibrium in the CO2 concentration between the seawater and atmosphere. The resulting flux from the atmosphere into the seawater replenishes the deficit in dissolved inorganic carbon due to seagrass photosynthesis. This replenishment may be complete (which maximizes carbon removal) or incomplete (which results in an inefficiency in carbon removal accounting).
- In theory, restoration (e.g., seed/seedling dispersal or planting) is the most likely mechanism for CDR, however, efforts to date are often unsuccessful[1] . Restoration propositions are further complicated by the outstanding questions around net carbon removal (especially under different climate change scenarios) and the extent of suitable locations.
- Restoration of hydrology, through reversing drainage or removing tidal restrictions, may assist in the recovery and expansion of existing seagrass beds[3] . This path may be met with more resistance (especially political and social) than other types of restoration efforts.
CDR Potential
Estimated Sequestration Potential: 0.007 – 0.025 Gt CO2e/year[4]
Sequestration Durability: Decades -- > 1,000 years[5] (Durability of sequestered carbon will depend on multiple variables and can vary greatly between individual ecosystems)
Challenges
- A 2023 review of all available literature on eelgrass (the dominant species of seagrass along the United States west coast) restoration projects in California, Oregon, and Washington found that of the 82 restoration projects, only 6 were published in peer-reviewed journals. This highlights the lack of data availability that can make assessing successes, failures, and best practices difficult[6] .
- Difficult to differentiate between autochthonous and allochthonous sources of carbon in sediments[7]
- There is substantial inorganic carbon in seagrasses which is derived from outside the seagrass bed[8] which in turn has sparked debate over whether seagrasses are ultimately a carbon sink or source.
- Seagrass restoration efforts are often unsuccessful[9]
- High variability in carbon burial rates[10]
- High cost associated with restoration efforts[11]
Key Knowledge Gaps
- Robust measurements of air-water CO2 exchange above seagrass beds
- Global extent of seagrasses (including future extent under changing climate)
- Causes of failed restoration projects
- What are the sources and fates of carbonates in seagrass beds?
First Order Priorities
*Adapted from the Environmental Defense Fund report on Coastal Natural Climate Solutions[12]
- Take additional measurements of air-sea gas exchange above seagrass beds*
- This will lead to the development of more robust predictive models to better understand CDR efficacy.
- Map the global extent of seagrasses and their likely future extent*
- This is likely to involve some combination of active remote sensing and field surveys as well as habitat suitability analyses.
- Mapping of likely future extent will involve considerations of future climate change impacts.
- Continue investigations into the causes of restoration project failures in seagrass systems*
- This effort will likely involve better monitoring, recording, and publication of data on restoration efforts.
- Improve the understanding of the sources and fate of carbonates in seagrass bed sediments*
- This includes the contributions to CO2 production both within seagrass beds and upstream of these ecosystems.
- Open systems where the open ocean is directly manipulated to enhance biological production, capture atmospheric CO2, and export the captured carbon to the deep ocean. In open systems, CO2 fixation is facilitated by the addition of limiting macronutrients (e.g., phosphorus, nitrogen, silica) and/or micronutrients (e.g., iron) to the ocean’s surface to augment biological production . Open system techniques accelerate natural processes already occurring in the ocean. Most approaches in the open ocean fall into the following two categories (some proposals that do not fit into these categories are also explored).
- Surface nutrient addition: the direct addition of nutrients (macro or micro) into ocean waters in situ to increase microalgal growth
- Nutrient upwelling: artificial upwelling of nutrient-rich deep ocean waters to the surface to increase microalgal growth
- Closed systems where inputs and growth conditions are controlled, and outputs (microalgae) are harvested within the confines of a pond or a photobioreactor. In closed systems, CO2 fixation is facilitated by the mixing of required inputs (sunlight, nutrients, CO2, water) and the introduction of microalgae culture with the intention of reproduction and continuous fixation in a contained system . This can be accomplished on shore in cultivation ponds or photobioreactors, or afloat in photobioreactors either stationary or towed at sea .
-
- Onshore: encompasses more established methods of microalgae cultivation, including photobioreactors, cultivation ponds, and hybrid onshore configurations. In these systems, all inputs are tightly controlled and regulated, and outputs must be directly managed through either storage or utilization of byproducts. While cultivation techniques are well-established and show high technological readiness, storage and utilization pathways remain underdeveloped and scale is a major consideration. Social and environmental risks for closed onshore systems are easier to monitor and mitigate due to the controlled nature of the system.
- Offshore: includes floating photobioreactors (PBRs) that are incorporated into a floating platform which can be stationary or towed behind a ship. In these systems, cultivation occurs in the photobioreactor, inputs are regulated, and outputs can be actively managed or directed. While at sea, these photobioreactors operate much like their onshore counterparts to cultivate microalgae, however nutrients and energy are provided by the ocean water and wave action, respectively. After microalgae are cultivated, they can be sunk into the deep ocean for sequestration or hauled to shore to be used as biomass. This is an area that has garnered much attention in startup communities (see this Y Combinator request for startups), however, little is available about the technologies in the open-sourced or peer reviewed literature.
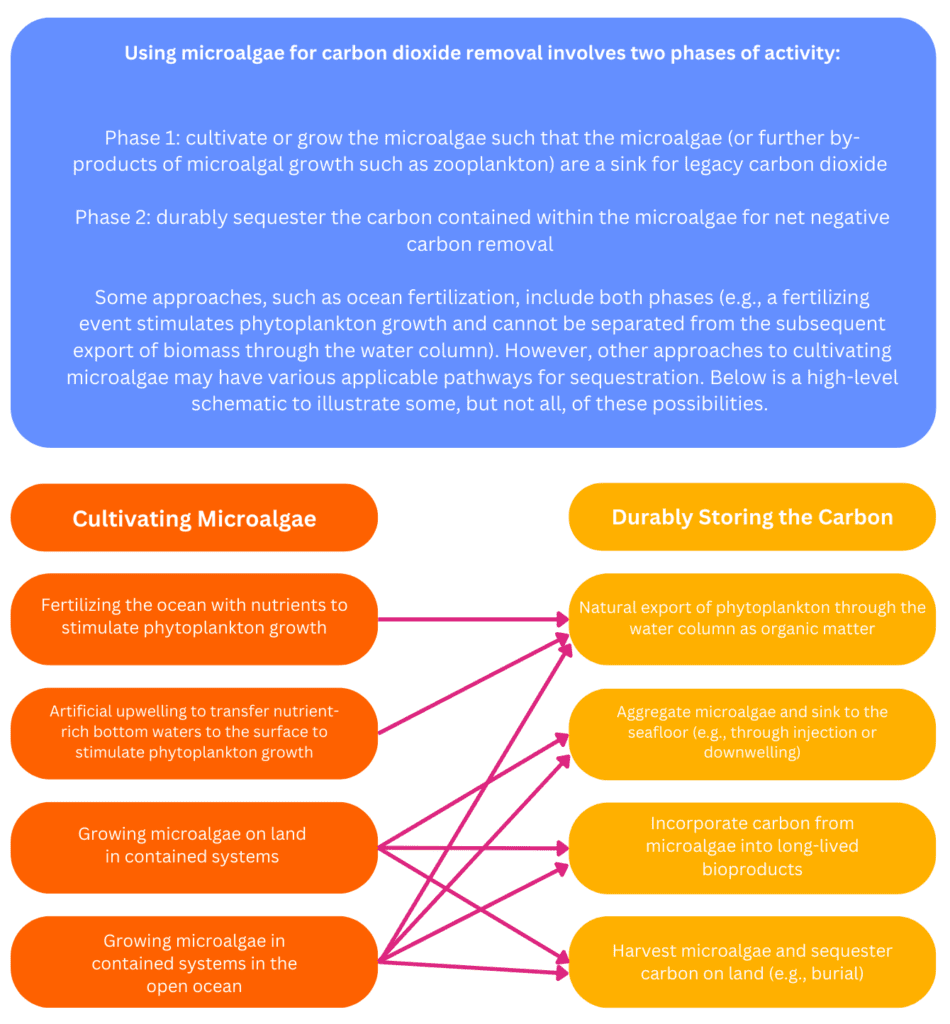
Environmental Co-Benefits of Coastal Vegetated Ecosystems
Restoration of coastal vegetated ecosystems can generate many benefits for surrounding marine ecosystems. While we are calling these “co-benefits” here, it should be noted that some of these benefits are so significant (while carbon sequestration feasibility and efficacy remain unknown) that these may in fact be the main benefits of restoration. (Note that there are significantly more co-benefits in coastal vegetated ecosystems than any of the other Ocean Visions’ marine CDR Road Maps.)
- Potential for ocean alkalinity enhancement (Fakhraee et al., 2023)
- Coastal protection against erosion (Barbier et al., 2011)
- Nutrient cycling (Mitra, 2019)
- Pollutant filtering
- Reducing biodiversity loss (NASEM, 2022)
- Restoring the role of marine organisms in the carbon cycle (NASEM, 2022)
- Protection against storm surge and inundation (Blankespoor et al., 2017; Liu et al., 2013; Zhang et al., 2012)
- Providing habitat and nurseries for a variety of marine organisms (Whitfield, 2017)
Restoration of coastal vegetated ecosystems can generate many benefits for surrounding marine ecosystems. While we are calling these “co-benefits” here, it should be noted that some of these benefits are so significant (while carbon sequestration feasibility and efficacy remain unknown) that these may in fact be the main benefits of restoration. (Note that there are significantly more co-benefits in coastal vegetated ecosystems than any of the other Ocean Visions’ marine CDR Road Maps.)
- Potential for ocean alkalinity enhancement (Fakhraee et al., 2023)
- Coastal protection against erosion (Barbier et al., 2011)
- Nutrient cycling (Mitra, 2019)
- Pollutant filtering
- Reducing biodiversity loss (NASEM, 2022)
- Restoring the role of marine organisms in the carbon cycle (NASEM, 2022)
- Protection against storm surge and inundation (Blankespoor et al., 2017; Liu et al., 2013; Zhang et al., 2012)
- Providing habitat and nurseries for a variety of marine organisms (Whitfield, 2017)
Restoration of coastal vegetated ecosystems can generate many benefits for surrounding marine ecosystems. While we are calling these “co-benefits” here, it should be noted that some of these benefits are so significant (while carbon sequestration feasibility and efficacy remain unknown) that these may in fact be the main benefits of restoration. (Note that there are significantly more co-benefits in coastal vegetated ecosystems than any of the other Ocean Visions’ marine CDR Road Maps.)
- Potential for ocean alkalinity enhancement
- Coastal protection against erosion
- Nutrient cycling
- Pollutant filtering
- Reducing biodiversity loss
- Restoring the role of marine organisms in the carbon cycle
- Protection against storm surge and inundation,,
- Providing habitat and nurseries for a variety of marine organisms
Restoration of coastal vegetated ecosystems can generate many benefits for surrounding marine ecosystems. While we are calling these “co-benefits” here, it should be noted that some of these benefits are so significant (while carbon sequestration feasibility and efficacy remain unknown) that these may in fact be the main benefits of restoration. (Note that there are significantly more co-benefits in coastal vegetated ecosystems than any of the other Ocean Visions’ marine CDR Road Maps.)
- Potential for ocean alkalinity enhancement[1]Fakhraee, Mojtaba, et al. “Ocean Alkalinity Enhancement through Restoration of Blue Carbon Ecosystems.” Nature Sustainability, 2023, https://doi.org/10.1038/s41893-023-01128-2.
- Coastal protection against erosion[2]Barbier, E. B. et al. The value of estuarine and coastal ecosystem services. Ecol. 697 Monogr. 81, 169–193 (2011).
- Nutrient cycling[3]Mitra, Abhijit. “Ecosystem Services of mangroves: An overview.” Mangrove Forests in India, 2019, pp. 1–32, https://doi.org/10.1007/978-3-030-20595-9_1.
- Pollutant filtering
- Reducing biodiversity loss[4]National Academies of Sciences, Engineering, and Medicine 2022. A Research Strategy for Ocean-based Carbon Dioxide Removal and Sequestration. Washington, DC: The National Academies Press. https://doi.org/10.17226/26278.
- Restoring the role of marine organisms in the carbon cycle[5]National Academies of Sciences, Engineering, and Medicine 2022. A Research Strategy for Ocean-based Carbon Dioxide Removal and Sequestration. Washington, DC: The National Academies Press. https://doi.org/10.17226/26278.
- Protection against storm surge and inundation[6]Blankespoor, B., Dasgupta, S. & Lange, GM. Mangroves as a protection from storm surges in a changing climate. Ambio 46, 478–491 (2017). https://doi.org/10.1007/s13280-016-0838-x ,[7]Liu, Huiqing, et al. “Numerical study of the sensitivity of mangroves in reducing storm surge and flooding to hurricane characteristics in southern Florida.” Continental Shelf Research, vol. 64, 2013, pp. 51–65, https://doi.org/10.1016/j.csr.2013.05.015. ,[8]Zhang, Keqi, et al. “The role of mangroves in attenuating storm surges.” Estuarine, Coastal and Shelf Science, vol. 102–103, 2012, pp. 11–23, https://doi.org/10.1016/j.ecss.2012.02.021.
- Providing habitat and nurseries for a variety of marine organisms[9]Whitfield, A.K. The role of seagrass meadows, mangrove forests, salt marshes and reed beds as nursery areas and food sources for fishes in estuaries. Rev Fish Biol Fisheries 27, 75–110 (2017). https://doi.org/10.1007/s11160-016-9454-x
Restoration of coastal vegetated ecosystems can generate many benefits for surrounding marine ecosystems. While we are calling these “co-benefits” here, it should be noted that some of these benefits are so significant (while carbon sequestration feasibility and efficacy remain unknown) that these may in fact be the main benefits of restoration. (Note that there are significantly more co-benefits in coastal vegetated ecosystems than any of the other Ocean Visions’ marine CDR Road Maps.)
- Potential for ocean alkalinity enhancement[1]Fakhraee, Mojtaba, et al. “Ocean Alkalinity Enhancement through Restoration of Blue Carbon Ecosystems.” Nature Sustainability, 2023, https://doi.org/10.1038/s41893-023-01128-2.
- Coastal protection against erosion[2]Barbier, E. B. et al. The value of estuarine and coastal ecosystem services. Ecol. 697 Monogr. 81, 169–193 (2011).
- Nutrient cycling[3]Mitra, Abhijit. “Ecosystem Services of mangroves: An overview.” Mangrove Forests in India, 2019, pp. 1–32, https://doi.org/10.1007/978-3-030-20595-9_1.
- Pollutant filtering
- Reducing biodiversity loss[4]National Academies of Sciences, Engineering, and Medicine 2022. A Research Strategy for Ocean-based Carbon Dioxide Removal and Sequestration. Washington, DC: The National Academies Press. https://doi.org/10.17226/26278.
- Restoring the role of marine organisms in the carbon cycle[5]National Academies of Sciences, Engineering, and Medicine 2022. A Research Strategy for Ocean-based Carbon Dioxide Removal and Sequestration. Washington, DC: The National Academies Press. https://doi.org/10.17226/26278.
- Protection against storm surge and inundation[6]Blankespoor, B., Dasgupta, S. & Lange, GM. Mangroves as a protection from storm surges in a changing climate. Ambio 46, 478–491 (2017). https://doi.org/10.1007/s13280-016-0838-x ,[7]Liu, Huiqing, et al. “Numerical study of the sensitivity of mangroves in reducing storm surge and flooding to hurricane characteristics in southern Florida.” Continental Shelf Research, vol. 64, 2013, pp. 51–65, https://doi.org/10.1016/j.csr.2013.05.015. ,[8]Zhang, Keqi, et al. “The role of mangroves in attenuating storm surges.” Estuarine, Coastal and Shelf Science, vol. 102–103, 2012, pp. 11–23, https://doi.org/10.1016/j.ecss.2012.02.021.
- Providing habitat and nurseries for a variety of marine organisms[9]Whitfield, A.K. The role of seagrass meadows, mangrove forests, salt marshes and reed beds as nursery areas and food sources for fishes in estuaries. Rev Fish Biol Fisheries 27, 75–110 (2017). https://doi.org/10.1007/s11160-016-9454-x
Restoration of coastal vegetated ecosystems can generate many benefits for surrounding marine ecosystems. While we are calling these “co-benefits” here, it should be noted that some of these benefits are so significant (while carbon sequestration feasibility and efficacy remain unknown) that these may in fact be the main benefits of restoration. (Note that there are significantly more co-benefits in coastal vegetated ecosystems than any of the other Ocean Visions’ marine CDR Road Maps.)
- Potential for ocean alkalinity enhancement[1]Fakhraee, Mojtaba, et al. “Ocean Alkalinity Enhancement through Restoration of Blue Carbon Ecosystems.” Nature Sustainability, 2023, https://doi.org/10.1038/s41893-023-01128-2.
- Coastal protection against erosion[2]Barbier, E. B. et al. The value of estuarine and coastal ecosystem services. Ecol. 697 Monogr. 81, 169–193 (2011).
- Nutrient cycling[3]Mitra, Abhijit. “Ecosystem Services of mangroves: An overview.” Mangrove Forests in India, 2019, pp. 1–32, https://doi.org/10.1007/978-3-030-20595-9_1.
- Pollutant filtering
- Reducing biodiversity loss[4]National Academies of Sciences, Engineering, and Medicine 2022. A Research Strategy for Ocean-based Carbon Dioxide Removal and Sequestration. Washington, DC: The National Academies Press. https://doi.org/10.17226/26278.
- Restoring the role of marine organisms in the carbon cycle[5]National Academies of Sciences, Engineering, and Medicine 2022. A Research Strategy for Ocean-based Carbon Dioxide Removal and Sequestration. Washington, DC: The National Academies Press. https://doi.org/10.17226/26278.
- Protection against storm surge and inundation[6]Blankespoor, B., Dasgupta, S. & Lange, GM. Mangroves as a protection from storm surges in a changing climate. Ambio 46, 478–491 (2017). https://doi.org/10.1007/s13280-016-0838-x ,[7]Liu, Huiqing, et al. “Numerical study of the sensitivity of mangroves in reducing storm surge and flooding to hurricane characteristics in southern Florida.” Continental Shelf Research, vol. 64, 2013, pp. 51–65, https://doi.org/10.1016/j.csr.2013.05.015. ,[8]Zhang, Keqi, et al. “The role of mangroves in attenuating storm surges.” Estuarine, Coastal and Shelf Science, vol. 102–103, 2012, pp. 11–23, https://doi.org/10.1016/j.ecss.2012.02.021.
- Providing habitat and nurseries for a variety of marine organisms[9]Whitfield, A.K. The role of seagrass meadows, mangrove forests, salt marshes and reed beds as nursery areas and food sources for fishes in estuaries. Rev Fish Biol Fisheries 27, 75–110 (2017). https://doi.org/10.1007/s11160-016-9454-x
- Open systems where the open ocean is directly manipulated to enhance biological production, capture atmospheric CO2, and export the captured carbon to the deep ocean. In open systems, CO2 fixation is facilitated by the addition of limiting macronutrients (e.g., phosphorus, nitrogen, silica) and/or micronutrients (e.g., iron) to the ocean’s surface to augment biological production . Open system techniques accelerate natural processes already occurring in the ocean. Most approaches in the open ocean fall into the following two categories (some proposals that do not fit into these categories are also explored).
- Surface nutrient addition: the direct addition of nutrients (macro or micro) into ocean waters in situ to increase microalgal growth
- Nutrient upwelling: artificial upwelling of nutrient-rich deep ocean waters to the surface to increase microalgal growth
- Closed systems where inputs and growth conditions are controlled, and outputs (microalgae) are harvested within the confines of a pond or a photobioreactor. In closed systems, CO2 fixation is facilitated by the mixing of required inputs (sunlight, nutrients, CO2, water) and the introduction of microalgae culture with the intention of reproduction and continuous fixation in a contained system . This can be accomplished on shore in cultivation ponds or photobioreactors, or afloat in photobioreactors either stationary or towed at sea .
-
- Onshore: encompasses more established methods of microalgae cultivation, including photobioreactors, cultivation ponds, and hybrid onshore configurations. In these systems, all inputs are tightly controlled and regulated, and outputs must be directly managed through either storage or utilization of byproducts. While cultivation techniques are well-established and show high technological readiness, storage and utilization pathways remain underdeveloped and scale is a major consideration. Social and environmental risks for closed onshore systems are easier to monitor and mitigate due to the controlled nature of the system.
- Offshore: includes floating photobioreactors (PBRs) that are incorporated into a floating platform which can be stationary or towed behind a ship. In these systems, cultivation occurs in the photobioreactor, inputs are regulated, and outputs can be actively managed or directed. While at sea, these photobioreactors operate much like their onshore counterparts to cultivate microalgae, however nutrients and energy are provided by the ocean water and wave action, respectively. After microalgae are cultivated, they can be sunk into the deep ocean for sequestration or hauled to shore to be used as biomass. This is an area that has garnered much attention in startup communities (see this Y Combinator request for startups), however, little is available about the technologies in the open-sourced or peer reviewed literature.
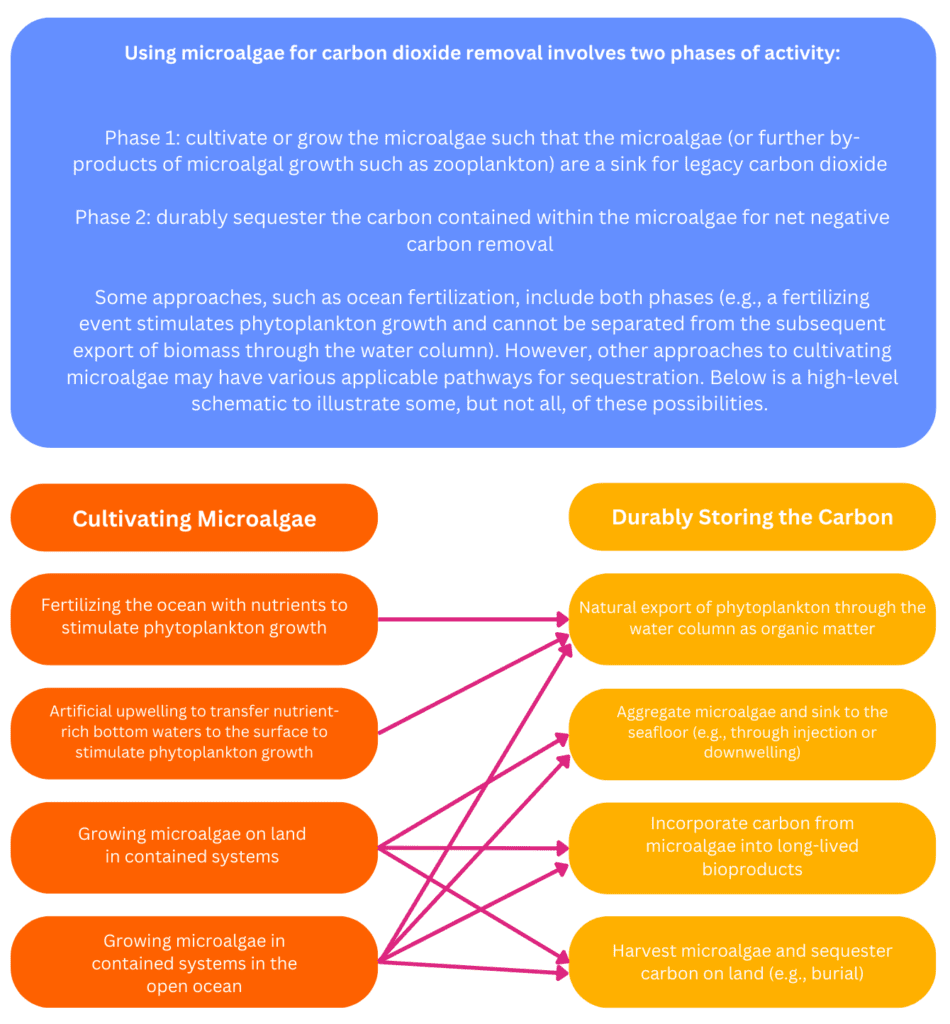
Environmental Risks of Coastal Vegetated Ecosystems
In contrast to other proposed marine CDR pathways, there are not many apparent environmental risks to utilizing coastal vegetated ecosystems for carbon dioxide removal. This is in part because coastal vegetated ecosystem pathways largely rely on restoration efforts which typically benefit local communities and surrounding ecosystems. However, proper restoration techniques which utilize the appropriate species (e.g., for mangrove replanting) are critical to ensuring no or limited negative environmental impacts. As with any marine CDR pathway, incremental scale-up with careful monitoring and verification of impacts will be critical to mitigate risks.
- Open systems where the open ocean is directly manipulated to enhance biological production, capture atmospheric CO2, and export the captured carbon to the deep ocean. In open systems, CO2 fixation is facilitated by the addition of limiting macronutrients (e.g., phosphorus, nitrogen, silica) and/or micronutrients (e.g., iron) to the ocean’s surface to augment biological production . Open system techniques accelerate natural processes already occurring in the ocean. Most approaches in the open ocean fall into the following two categories (some proposals that do not fit into these categories are also explored).
- Surface nutrient addition: the direct addition of nutrients (macro or micro) into ocean waters in situ to increase microalgal growth
- Nutrient upwelling: artificial upwelling of nutrient-rich deep ocean waters to the surface to increase microalgal growth
- Closed systems where inputs and growth conditions are controlled, and outputs (microalgae) are harvested within the confines of a pond or a photobioreactor. In closed systems, CO2 fixation is facilitated by the mixing of required inputs (sunlight, nutrients, CO2, water) and the introduction of microalgae culture with the intention of reproduction and continuous fixation in a contained system . This can be accomplished on shore in cultivation ponds or photobioreactors, or afloat in photobioreactors either stationary or towed at sea .
-
- Onshore: encompasses more established methods of microalgae cultivation, including photobioreactors, cultivation ponds, and hybrid onshore configurations. In these systems, all inputs are tightly controlled and regulated, and outputs must be directly managed through either storage or utilization of byproducts. While cultivation techniques are well-established and show high technological readiness, storage and utilization pathways remain underdeveloped and scale is a major consideration. Social and environmental risks for closed onshore systems are easier to monitor and mitigate due to the controlled nature of the system.
- Offshore: includes floating photobioreactors (PBRs) that are incorporated into a floating platform which can be stationary or towed behind a ship. In these systems, cultivation occurs in the photobioreactor, inputs are regulated, and outputs can be actively managed or directed. While at sea, these photobioreactors operate much like their onshore counterparts to cultivate microalgae, however nutrients and energy are provided by the ocean water and wave action, respectively. After microalgae are cultivated, they can be sunk into the deep ocean for sequestration or hauled to shore to be used as biomass. This is an area that has garnered much attention in startup communities (see this Y Combinator request for startups), however, little is available about the technologies in the open-sourced or peer reviewed literature.
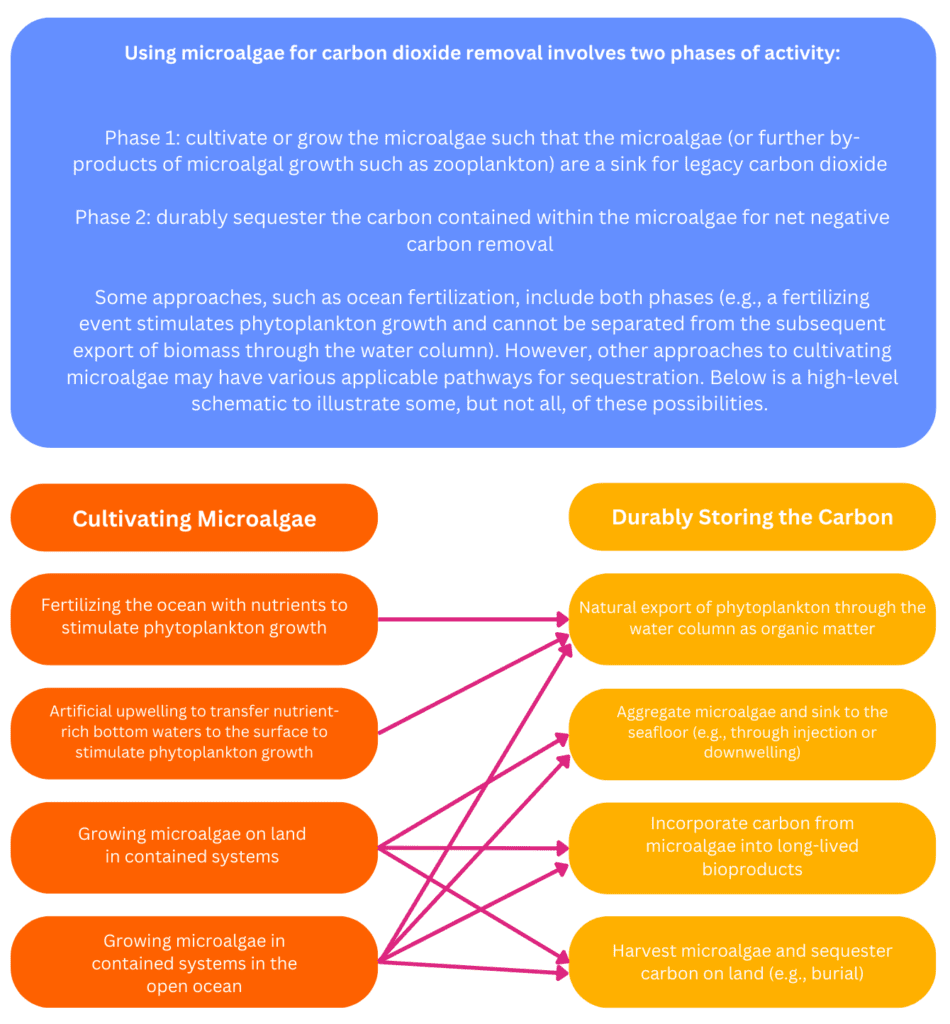
First-Order Priorities Common to All Coastal Vegetated Ecosystems
- Develop accessible (economically and geographically) sensors and technologies to measure greenhouse gas exchanges and fluxes both temporally and spatially at high enough resolution to create predictive models for net carbon sequestration and durability.
- Create models that account for future impacts/effects of climate change and land-use change (e.g., coastal squeeze)
- Create new frameworks (e.g., seascape (Huxham et al., 2018) or watershed approaches) both for research and financial mechanisms that account for intersystem linkages and take a more holistic approach to measuring carbon associated with coastal vegetated ecosystems.
- Currently, ecosystems are considered and studied individually which can cause issues when thinking about the movement of carbon between systems
- Investigate and characterize the historical or pre-industrial ecosystem locations and ranges (which in many cases are currently unknown) in order to establish baselines for restoration projects.
- In some instances, these prior states may need to be derived from historical sources including more traditional data sets and non-traditional data sets like paintings (Duarte et al., 2020).
- Develop accessible (economically and geographically) sensors and technologies to measure greenhouse gas exchanges and fluxes both temporally and spatially at high enough resolution to create predictive models for net carbon sequestration and durability.
- Create models that account for future impacts/effects of climate change and land-use change (e.g., coastal squeeze)
- Create new frameworks (e.g., seascape (Huxham et al., 2018) or watershed approaches) both for research and financial mechanisms that account for intersystem linkages and take a more holistic approach to measuring carbon associated with coastal vegetated ecosystems.
- Currently, ecosystems are considered and studied individually which can cause issues when thinking about the movement of carbon between systems
- Investigate and characterize the historical or pre-industrial ecosystem locations and ranges (which in many cases are currently unknown) in order to establish baselines for restoration projects.
- In some instances, these prior states may need to be derived from historical sources including more traditional data sets and non-traditional data sets like paintings (Duarte et al., 2020).
- Develop accessible (economically and geographically) sensors and technologies to measure greenhouse gas exchanges and fluxes both temporally and spatially at high enough resolution to create predictive models for net carbon sequestration and durability.
- Create models that account for future impacts/effects of climate change and land-use change (e.g., coastal squeeze)
- Create new frameworks (e.g., seascape or watershed approaches) both for research and financial mechanisms that account for intersystem linkages and take a more holistic approach to measuring carbon associated with coastal vegetated ecosystems.
- Currently, ecosystems are considered and studied individually which can cause issues when thinking about the movement of carbon between systems
- Investigate and characterize the historical or pre-industrial ecosystem locations and ranges (which in many cases are currently unknown) in order to establish baselines for restoration projects.
- In some instances, these prior states may need to be derived from historical sources including more traditional data sets and non-traditional data sets like paintings.
- Develop accessible (economically and geographically) sensors and technologies to measure greenhouse gas exchanges and fluxes both temporally and spatially at high enough resolution to create predictive models for net carbon sequestration and durability.
- Create models that account for future impacts/effects of climate change and land-use change (e.g., coastal squeeze)
- Create new frameworks (e.g., seascape[1]Huxham, M., et al. “Carbon in the coastal seascape: How interactions between mangrove forests, seagrass meadows and tidal marshes influence carbon storage.” Current Forestry Reports, vol. 4, no. 2, 2018, pp. 101–110, https://doi.org/10.1007/s40725-018-0077-4.
or watershed approaches) both for research and financial mechanisms that account for intersystem linkages and take a more holistic approach to measuring coastal blue carbon.
- Currently, ecosystems are considered and studied individually which can cause issues when thinking about the movement of carbon between systems
- Investigate and characterize the historical or pre-industrial ecosystem locations and ranges (which in many cases are currently unknown) in order to establish baselines for restoration projects.
- Develop accessible (economically and geographically) sensors and technologies to measure greenhouse gas exchanges and fluxes both temporally and spatially at high enough resolution to create predictive models for net carbon sequestration and durability.
- Create models that account for future impacts/effects of climate change and land-use change (e.g., coastal squeeze)
- Create new frameworks (e.g., seascape[1]Huxham, M., et al. “Carbon in the coastal seascape: How interactions between mangrove forests, seagrass meadows and tidal marshes influence carbon storage.” Current Forestry Reports, vol. 4, no. 2, 2018, pp. 101–110, https://doi.org/10.1007/s40725-018-0077-4.
or watershed approaches) both for research and financial mechanisms that account for intersystem linkages and take a more holistic approach to measuring coastal blue carbon.
- Currently, ecosystems are considered and studied individually which can cause issues when thinking about the movement of carbon between systems
- Investigate and characterize the historical or pre-industrial ecosystem locations and ranges (which in many cases are currently unknown) in order to establish baselines for restoration projects.
- Develop accessible (economically and geographically) sensors and technologies to measure greenhouse gas exchanges and fluxes both temporally and spatially at high enough resolution to create predictive models for net carbon sequestration and durability.
- Create models that account for future impacts/effects of climate change and land-use change (e.g., coastal squeeze)
- Create new frameworks (e.g., seascape[1]Huxham, M., et al. “Carbon in the coastal seascape: How interactions between mangrove forests, seagrass meadows and tidal marshes influence carbon storage.” Current Forestry Reports, vol. 4, no. 2, 2018, pp. 101–110, https://doi.org/10.1007/s40725-018-0077-4.
or watershed approaches) both for research and financial mechanisms that account for intersystem linkages and take a more holistic approach to measuring coastal blue carbon.
- Currently, ecosystems are considered and studied individually which can cause issues when thinking about the movement of carbon between systems
- Investigate and characterize the historical or pre-industrial ecosystem locations and ranges (which in many cases are currently unknown) in order to establish baselines for restoration projects.
- Open systems where the open ocean is directly manipulated to enhance biological production, capture atmospheric CO2, and export the captured carbon to the deep ocean. In open systems, CO2 fixation is facilitated by the addition of limiting macronutrients (e.g., phosphorus, nitrogen, silica) and/or micronutrients (e.g., iron) to the ocean’s surface to augment biological production . Open system techniques accelerate natural processes already occurring in the ocean. Most approaches in the open ocean fall into the following two categories (some proposals that do not fit into these categories are also explored).
- Surface nutrient addition: the direct addition of nutrients (macro or micro) into ocean waters in situ to increase microalgal growth
- Nutrient upwelling: artificial upwelling of nutrient-rich deep ocean waters to the surface to increase microalgal growth
- Closed systems where inputs and growth conditions are controlled, and outputs (microalgae) are harvested within the confines of a pond or a photobioreactor. In closed systems, CO2 fixation is facilitated by the mixing of required inputs (sunlight, nutrients, CO2, water) and the introduction of microalgae culture with the intention of reproduction and continuous fixation in a contained system . This can be accomplished on shore in cultivation ponds or photobioreactors, or afloat in photobioreactors either stationary or towed at sea .
-
- Onshore: encompasses more established methods of microalgae cultivation, including photobioreactors, cultivation ponds, and hybrid onshore configurations. In these systems, all inputs are tightly controlled and regulated, and outputs must be directly managed through either storage or utilization of byproducts. While cultivation techniques are well-established and show high technological readiness, storage and utilization pathways remain underdeveloped and scale is a major consideration. Social and environmental risks for closed onshore systems are easier to monitor and mitigate due to the controlled nature of the system.
- Offshore: includes floating photobioreactors (PBRs) that are incorporated into a floating platform which can be stationary or towed behind a ship. In these systems, cultivation occurs in the photobioreactor, inputs are regulated, and outputs can be actively managed or directed. While at sea, these photobioreactors operate much like their onshore counterparts to cultivate microalgae, however nutrients and energy are provided by the ocean water and wave action, respectively. After microalgae are cultivated, they can be sunk into the deep ocean for sequestration or hauled to shore to be used as biomass. This is an area that has garnered much attention in startup communities (see this Y Combinator request for startups), however, little is available about the technologies in the open-sourced or peer reviewed literature.
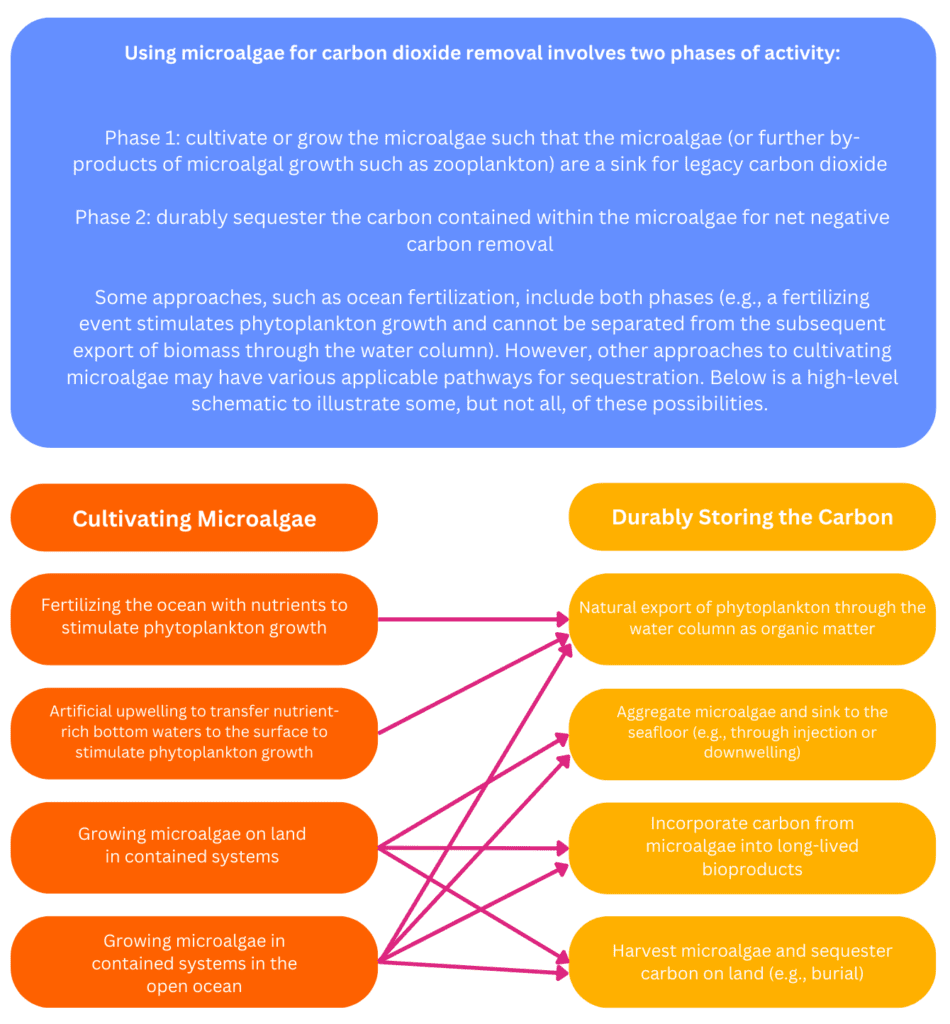
Current Financing Landscape & Common Challenges
Historically, a few financing streams have been developed to support the many benefits of coastal vegetated ecosystem restoration projects. These include nutrient remediation markets (see The Wetlands Initiative), biodiversity mitigation banks, and government subsidies. In order for restoration projects for CDR to be realized at scale, there needs to be a financing mechanism(s) in place that can also scale appropriately. One such mechanism that is currently being explored is the carbon credit for use on the carbon market. A carbon credit is a tradeable certificate that represents one metric tonne of CO2 equivalent. These credits can be generated from an array of activities, such as planting trees, so long as a measurable amount of CO2 equivalent is being removed or reduced. For the purpose of marine CDR, we will focus only on credits for CO2 removal. Carbon credits are traded on carbon markets which can either be voluntary or compliance. Compliance markets can either be national or international government-regulated programs whereas voluntary markets trade internationally and supply carbon credits to individuals and companies. There is no single set of rules that regulates carbon markets, however there are bodies that provide recommendations and set standards or best practices.
Current Landscape
- Verra: In 2020 Verra, a standard for certifying carbon credits, released its first blue carbon conservation methodology, an update to the VCS REDD+ Methodology Framework. Verra has also formed a blue carbon Working Group (2020) that explores opportunities for coastal wetland restoration and conservation as well as developed the Wetland Restoration and Conservation Requirements. Addressing blue carbon in the open ocean, Verra created an Ocean Carbon Working Group as well as the Seascape Carbon Initiative (co-led by Verra, Silvestrum Climate Associates, Blue Marine Foundation, the blue carbon Initiative, and Oceans 2050).
- Gold Standard: Gold Standard is a carbon offset standard for non-governmental emission reductions projects. Gold Standard has an approved methodology for the certification of mangrove projects and are exploring other blue carbon opportunities.
- Plan Vivo: Plan Vivo is a certification body that certifies projects against the Plan Vivo Standard, a framework for community and smallholder land-use and forestry projects. In 2014, Plan Vivo certified the world’s first blue carbon project, Mikoko Pamoja (Kenya), for mangrove conservation.
- Fair Carbon: Fair Carbon supports the development of blue Carbon projects by increasing the accessibility of information and transparency. This includes their Global Blue Carbon Projects map which allows access to all publicly registered blue carbon projects across multiple voluntary carbon market registries. They also have a number of other resources around carbon credits for blue carbon.
- Sustainable Development Mechanism (formerly the Clean Development Mechanism): This is a compliance market designed for shorter-term emissions credits.
- Nationally Determined Contributions (NDCs): These are the climate actions detailed and executed by countries participating in the Paris Agreement and serve as the primary mechanism for meeting the goals set out in the Paris Agreement. The restoration of blue carbon ecosystems may help mitigate and adapt to climate change, however, their integration into Nationally Determined Contributions remains underdeveloped (Dencer-Brown et al., 2022). A 2022 report from thirty-three experts highlights the need for “genuine participation by communities, inclusive project governance, integration of local work into national policies and practices, sustaining livelihoods and income (for example through the voluntary carbon market and/or national Payment for Ecosystem Services and other types of financial compensation systems) and simplification of carbon accounting and verification methodologies to lower barriers to entry (Dencer-Brown et al., 2022)” in order for blue carbon projects to be responsibly and successfully integrated into Nationally Determined Contributions.
- New efforts to improve the quality of carbon credits and the voluntary carbon market are being discussed and developed (See EDF, 2023). The integrity of each carbon credit will be critical to enable the carbon market to address climate change. New guidance has been released in 2023 to ensure carbon credits deliver on their claims. The Integrity Council for the Voluntary Carbon Market released its Core Carbon Principles that set a global threshold to define what a high-integrity carbon credit is.
Challenges
- Verification of the amount of carbon sequestered and its durability can be extremely challenging across coastal vegetated ecosystems but is necessary for effective (in terms of mitigating climate change) carbon markets.
- Ensuring fairness and equity in benefits distribution
Current Landscape
- Verra: In 2020 Verra, a standard for certifying carbon credits, released its first blue carbon conservation methodology, an update to the VCS REDD+ Methodology Framework. Verra has also formed a blue carbon Working Group (2020) that explores opportunities for coastal wetland restoration and conservation as well as developed the Wetland Restoration and Conservation Requirements. Addressing blue carbon in the open ocean, Verra created an Ocean Carbon Working Group as well as the Seascape Carbon Initiative (co-led by Verra, Silvestrum Climate Associates, Blue Marine Foundation, the blue carbon Initiative, and Oceans 2050).
- Gold Standard: Gold Standard is a carbon offset standard for non-governmental emission reductions projects. Gold Standard has an approved methodology for the certification of mangrove projects and are exploring other blue carbon opportunities.
- Plan Vivo: Plan Vivo is a certification body that certifies projects against the Plan Vivo Standard, a framework for community and smallholder land-use and forestry projects. In 2014, Plan Vivo certified the world’s first blue carbon project, Mikoko Pamoja (Kenya), for mangrove conservation.
- Fair Carbon: Fair Carbon supports the development of blue Carbon projects by increasing the accessibility of information and transparency. This includes their Global Blue Carbon Projects map which allows access to all publicly registered blue carbon projects across multiple voluntary carbon market registries. They also have a number of other resources around carbon credits for blue carbon.
- Sustainable Development Mechanism (formerly the Clean Development Mechanism): This is a compliance market designed for shorter-term emissions credits.
- Nationally Determined Contributions (NDCs): These are the climate actions detailed and executed by countries participating in the Paris Agreement and serve as the primary mechanism for meeting the goals set out in the Paris Agreement. The restoration of blue carbon ecosystems may help mitigate and adapt to climate change, however, their integration into Nationally Determined Contributions remains underdeveloped (Dencer-Brown et al., 2022). A 2022 report from thirty-three experts highlights the need for “genuine participation by communities, inclusive project governance, integration of local work into national policies and practices, sustaining livelihoods and income (for example through the voluntary carbon market and/or national Payment for Ecosystem Services and other types of financial compensation systems) and simplification of carbon accounting and verification methodologies to lower barriers to entry (Dencer-Brown et al., 2022)” in order for blue carbon projects to be responsibly and successfully integrated into Nationally Determined Contributions.
- New efforts to improve the quality of carbon credits and the voluntary carbon market are being discussed and developed (See EDF, 2023). The integrity of each carbon credit will be critical to enable the carbon market to address climate change. New guidance has been released in 2023 to ensure carbon credits deliver on their claims. The Integrity Council for the Voluntary Carbon Market released its Core Carbon Principles that set a global threshold to define what a high-integrity carbon credit is.
Challenges
- Verification of the amount of carbon sequestered and its durability can be extremely challenging across coastal vegetated ecosystems but is necessary for effective (in terms of mitigating climate change) carbon markets.
- Ensuring fairness and equity in benefits distribution
Current Landscape
- Verra: In 2020 Verra, a standard for certifying carbon credits, released its first blue carbon conservation methodology, an update to the VCS REDD+ Methodology Framework. Verra has also formed a blue carbon Working Group (2020) that explores opportunities for coastal wetland restoration and conservation as well as developed the Wetland Restoration and Conservation Requirements. Addressing blue carbon in the open ocean, Verra created an Ocean Carbon Working Group as well as the Seascape Carbon Initiative (co-led by Verra, Silvestrum Climate Associates, Blue Marine Foundation, the blue carbon Initiative, and Oceans 2050)[4]https://verra.org/programs/verified-carbon-standard/area-of-focus-blue-carbon/ .
- Gold Standard: Gold Standard is a carbon offset standard for non-governmental emission reductions projects. Gold Standard has an approved methodology for the certification of mangrove projects and are exploring other blue carbon opportunities.
- Plan Vivo: Plan Vivo is a certification body that certifies projects against the Plan Vivo Standard, a framework for community and smallholder land-use and forestry projects. In 2014, Plan Vivo certified the world’s first blue carbon project, Mikoko Pamoja (Kenya), for mangrove conservation[5]https://www.planvivo.org/news/our-statement-on-blue-carbon .
- Fair Carbon: Fair Carbon supports the development of blue Carbon projects by increasing the accessibility of information and transparency[6]https://faircarbon.org/ . This includes their Global Blue Carbon Projects map which allows access to all publicly registered blue carbon projects across multiple voluntary carbon market registries. They also have a number of other resources around carbon credits for blue carbon.
- Sustainable Development Mechanism (formerly the Clean Development Mechanism): This is a compliance market designed for shorter-term emissions credits.
- Nationally Determined Contributions (NDCs): These are the climate actions detailed and executed by countries participating in the Paris Agreement and serve as the primary mechanism for meeting the goals set out in the Paris Agreement. The restoration of blue carbon ecosystems may help mitigate and adapt to climate change, however their integration into Nationally Determined Contributions remains underdeveloped[7]Dencer-Brown, Amrit Melissa, et al. “Integrating Blue: How Do We Make Nationally Determined Contributions Work for Both Blue Carbon and Local Coastal Communities?” Ambio, vol. 51, no. 9, 2022, pp. 1978–1993, https://doi.org/10.1007/s13280-022-01723-1. . A 2022 report from thirty three experts highlights the need for “genuine participation by communities, inclusive project governance, integration of local work into national policies and practices, sustaining livelihoods and income (for example through the voluntary carbon market and/or national Payment for Ecosystem Services and other types of financial compensation systems) and simplification of carbon accounting and verification methodologies to lower barriers to entry[8]Dencer-Brown, Amrit Melissa, et al. “Integrating Blue: How Do We Make Nationally Determined Contributions Work for Both Blue Carbon and Local Coastal Communities?” Ambio, vol. 51, no. 9, 2022, pp. 1978–1993, https://doi.org/10.1007/s13280-022-01723-1. ” in order for blue carbon projects to be responsibly and successfully integrated into Nationally Determined Contributions.
- New efforts to improve the quality of carbon credits and the voluntary carbon market are being discussed and developed[9]https://blogs.edf.org/climate411/2023/08/01/navigating-the-core-carbon-principles-and-the-landscape-of-guidance-toward-a-high-integrity-carbon-market/ . The integrity of each carbon credit will be critical to enable the carbon market to address climate change. New guidance has been released in 2023 to ensure carbon credits deliver on their claims. The Integrity Council for the Voluntary Carbon Market released its Core Carbon Principles[10]https://icvcm.org/the-core-carbon-principles/ that set a global threshold to define what a high-integrity carbon credit is.
Challenges
- Verification of the amount of carbon sequestered and its durability can be extremely challenging across coastal vegetated ecosystems but is necessary for effective (in terms of mitigating climate change) carbon markets.
- Ensuring fairness and equity in benefits distribution
Current Landscape
- Verra: In 2020 Verra, a standard for certifying carbon credits, released its first blue carbon conservation methodology, an update to the VCS REDD+ Methodology Framework. Verra has also formed a blue carbon Working Group (2020) that explores opportunities for coastal wetland restoration and conservation as well as developed the Wetland Restoration and Conservation Requirements. Addressing blue carbon in the open ocean, Verra created an Ocean Carbon Working Group as well as the Seascape Carbon Initiative (co-led by Verra, Silvestrum Climate Associates, Blue Marine Foundation, the blue carbon Initiative, and Oceans 2050).
- Gold Standard: Gold Standard is a carbon offset standard for non-governmental emission reductions projects. Gold Standard has an approved methodology for the certification of mangrove projects and are exploring other blue carbon opportunities.
- Plan Vivo: Plan Vivo is a certification body that certifies projects against the Plan Vivo Standard, a framework for community and smallholder land-use and forestry projects. In 2014, Plan Vivo certified the world’s first blue carbon project, Mikoko Pamoja (Kenya), for mangrove conservation.
- Fair Carbon: Fair Carbon supports the development of blue Carbon projects by increasing the accessibility of information and transparency. This includes their Global Blue Carbon Projects map which allows access to all publicly registered blue carbon projects across multiple voluntary carbon market registries. They also have a number of other resources around carbon credits for blue carbon.
- Sustainable Development Mechanism (formerly the Clean Development Mechanism): This is a compliance market designed for shorter-term emissions credits.
- Nationally Determined Contributions (NDCs): These are the climate actions detailed and executed by countries participating in the Paris Agreement and serve as the primary mechanism for meeting the goals set out in the Paris Agreement. The restoration of blue carbon ecosystems may help mitigate and adapt to climate change, however their integration into Nationally Determined Contributions remains underdeveloped. A 2022 report from thirty three experts highlights the need for “genuine participation by communities, inclusive project governance, integration of local work into national policies and practices, sustaining livelihoods and income (for example through the voluntary carbon market and/or national Payment for Ecosystem Services and other types of financial compensation systems) and simplification of carbon accounting and verification methodologies to lower barriers to entry” in order for blue carbon projects to be responsibly and successfully integrated into Nationally Determined Contributions.
- New efforts to improve the quality of carbon credits and the voluntary carbon market are being discussed and developed. The integrity of each carbon credit will be critical to enable the carbon market to address climate change. New guidance has been released in 2023 to ensure carbon credits deliver on their claims. The Integrity Council for the Voluntary Carbon Market released its Core Carbon Principles that set a global threshold to define what a high-integrity carbon credit is.
Challenges
- Verification of the amount of carbon sequestered and its durability can be extremely challenging across coastal vegetated ecosystems but is necessary for effective (in terms of mitigating climate change) carbon markets.
- Ensuring fairness and equity in benefits distribution
Current Landscape
- Verra: In 2020 Verra, a standard for certifying carbon credits, released its first blue carbon conservation methodology, an update to the VCS REDD+ Methodology Framework. Verra has also formed a blue carbon Working Group (2020) that explores opportunities for coastal wetland restoration and conservation as well as developed the Wetland Restoration and Conservation Requirements. Addressing blue carbon in the open ocean, Verra created an Ocean Carbon Working Group as well as the Seascape Carbon Initiative (co-led by Verra, Silvestrum Climate Associates, Blue Marine Foundation, the blue carbon Initiative, and Oceans 2050).
- Gold Standard: Gold Standard is a carbon offset standard for non-governmental emission reductions projects. Gold Standard has an approved methodology for the certification of mangrove projects and are exploring other blue carbon opportunities.
- Plan Vivo: Plan Vivo is a certification body that certifies projects against the Plan Vivo Standard, a framework for community and smallholder land-use and forestry projects. In 2014, Plan Vivo certified the world’s first blue carbon project, Mikoko Pamoja (Kenya), for mangrove conservation.
- Fair Carbon: Fair Carbon supports the development of blue Carbon projects by increasing the accessibility of information and transparency. This includes their Global Blue Carbon Projects map which allows access to all publicly registered blue carbon projects across multiple voluntary carbon market registries. They also have a number of other resources around carbon credits for blue carbon.
- Sustainable Development Mechanism (formerly the Clean Development Mechanism): This is a compliance market designed for shorter-term emissions credits.
- Nationally Determined Contributions (NDCs): These are the climate actions detailed and executed by countries participating in the Paris Agreement and serve as the primary mechanism for meeting the goals set out in the Paris Agreement. The restoration of blue carbon ecosystems may help mitigate and adapt to climate change, however their integration into Nationally Determined Contributions remains underdeveloped. A 2022 report from thirty three experts highlights the need for “genuine participation by communities, inclusive project governance, integration of local work into national policies and practices, sustaining livelihoods and income (for example through the voluntary carbon market and/or national Payment for Ecosystem Services and other types of financial compensation systems) and simplification of carbon accounting and verification methodologies to lower barriers to entry” in order for blue carbon projects to be responsibly and successfully integrated into Nationally Determined Contributions.
- New efforts to improve the quality of carbon credits and the voluntary carbon market are being discussed and developed. The integrity of each carbon credit will be critical to enable the carbon market to address climate change. New guidance has been released in 2023 to ensure carbon credits deliver on their claims. The Integrity Council for the Voluntary Carbon Market released its Core Carbon Principles that set a global threshold to define what a high-integrity carbon credit is.
Challenges
- Verification of the amount of carbon sequestered and its durability can be extremely challenging across coastal vegetated ecosystems but is necessary for effective (in terms of mitigating climate change) carbon markets.
- Ensuring fairness and equity in benefits distribution
Current Landscape
- Verra: In 2020 Verra, a standard for certifying carbon credits, released its first blue carbon conservation methodology, an update to the VCS REDD+ Methodology Framework. Verra has also formed a blue carbon Working Group (2020) that explores opportunities for coastal wetland restoration and conservation as well as developed the Wetland Restoration and Conservation Requirements. Addressing blue carbon in the open ocean, Verra created an Ocean Carbon Working Group as well as the Seascape Carbon Initiative (co-led by Verra, Silvestrum Climate Associates, Blue Marine Foundation, the blue carbon Initiative, and Oceans 2050)[4]https://verra.org/programs/verified-carbon-standard/area-of-focus-blue-carbon/ .
- Gold Standard: Gold Standard is a carbon offset standard for non-governmental emission reductions projects. Gold Standard has an approved methodology for the certification of mangrove projects and are exploring other blue carbon opportunities.
- Plan Vivo: Plan Vivo is a certification body that certifies projects against the Plan Vivo Standard, a framework for community and smallholder land-use and forestry projects. In 2014, Plan Vivo certified the world’s first blue carbon project, Mikoko Pamoja (Kenya), for mangrove conservation[5]https://www.planvivo.org/news/our-statement-on-blue-carbon .
- Fair Carbon: Fair Carbon supports the development of blue Carbon projects by increasing the accessibility of information and transparency[6]https://faircarbon.org/ . This includes their Global Blue Carbon Projects map which allows access to all publicly registered blue carbon projects across multiple voluntary carbon market registries. They also have a number of other resources around carbon credits for blue carbon.
- Sustainable Development Mechanism (formerly the Clean Development Mechanism): This is a compliance market designed for shorter-term emissions credits.
- Nationally Determined Contributions (NDCs): These are the climate actions detailed and executed by countries participating in the Paris Agreement and serve as the primary mechanism for meeting the goals set out in the Paris Agreement. The restoration of blue carbon ecosystems may help mitigate and adapt to climate change, however their integration into Nationally Determined Contributions remains underdeveloped[7]Dencer-Brown, Amrit Melissa, et al. “Integrating Blue: How Do We Make Nationally Determined Contributions Work for Both Blue Carbon and Local Coastal Communities?” Ambio, vol. 51, no. 9, 2022, pp. 1978–1993, https://doi.org/10.1007/s13280-022-01723-1. . A 2022 report from thirty three experts highlights the need for “genuine participation by communities, inclusive project governance, integration of local work into national policies and practices, sustaining livelihoods and income (for example through the voluntary carbon market and/or national Payment for Ecosystem Services and other types of financial compensation systems) and simplification of carbon accounting and verification methodologies to lower barriers to entry[8]Dencer-Brown, Amrit Melissa, et al. “Integrating Blue: How Do We Make Nationally Determined Contributions Work for Both Blue Carbon and Local Coastal Communities?” Ambio, vol. 51, no. 9, 2022, pp. 1978–1993, https://doi.org/10.1007/s13280-022-01723-1. ” in order for blue carbon projects to be responsibly and successfully integrated into Nationally Determined Contributions.
- New efforts to improve the quality of carbon credits and the voluntary carbon market are being discussed and developed[9]https://blogs.edf.org/climate411/2023/08/01/navigating-the-core-carbon-principles-and-the-landscape-of-guidance-toward-a-high-integrity-carbon-market/ . The integrity of each carbon credit will be critical to enable the carbon market to address climate change. New guidance has been released in 2023 to ensure carbon credits deliver on their claims. The Integrity Council for the Voluntary Carbon Market released its Core Carbon Principles[10]https://icvcm.org/the-core-carbon-principles/ that set a global threshold to define what a high-integrity carbon credit is.
Challenges
- Verification of the amount of carbon sequestered and its durability can be extremely challenging across coastal vegetated ecosystems but is necessary for effective (in terms of mitigating climate change) carbon markets.
- Ensuring fairness and equity in benefits distribution
Current Landscape
- Verra: In 2020 Verra, a standard for certifying carbon credits, released its first blue carbon conservation methodology, an update to the VCS REDD+ Methodology Framework. Verra has also formed a blue carbon Working Group (2020) that explores opportunities for coastal wetland restoration and conservation as well as developed the Wetland Restoration and Conservation Requirements. Addressing blue carbon in the open ocean, Verra created an Ocean Carbon Working Group as well as the Seascape Carbon Initiative (co-led by Verra, Silvestrum Climate Associates, Blue Marine Foundation, the blue carbon Initiative, and Oceans 2050)[4]https://verra.org/programs/verified-carbon-standard/area-of-focus-blue-carbon/ .
- Gold Standard: Gold Standard is a carbon offset standard for non-governmental emission reductions projects. Gold Standard has an approved methodology for the certification of mangrove projects and are exploring other blue carbon opportunities.
- Plan Vivo: Plan Vivo is a certification body that certifies projects against the Plan Vivo Standard, a framework for community and smallholder land-use and forestry projects. In 2014, Plan Vivo certified the world’s first blue carbon project, Mikoko Pamoja (Kenya), for mangrove conservation[5]https://www.planvivo.org/news/our-statement-on-blue-carbon .
- Fair Carbon: Fair Carbon supports the development of blue Carbon projects by increasing the accessibility of information and transparency[6]https://faircarbon.org/ . This includes their Global Blue Carbon Projects map which allows access to all publicly registered blue carbon projects across multiple voluntary carbon market registries. They also have a number of other resources around carbon credits for blue carbon.
- Sustainable Development Mechanism (formerly the Clean Development Mechanism): This is a compliance market designed for shorter-term emissions credits.
- Nationally Determined Contributions (NDCs): These are the climate actions detailed and executed by countries participating in the Paris Agreement and serve as the primary mechanism for meeting the goals set out in the Paris Agreement. The restoration of blue carbon ecosystems may help mitigate and adapt to climate change, however their integration into Nationally Determined Contributions remains underdeveloped[7]Dencer-Brown, Amrit Melissa, et al. “Integrating Blue: How Do We Make Nationally Determined Contributions Work for Both Blue Carbon and Local Coastal Communities?” Ambio, vol. 51, no. 9, 2022, pp. 1978–1993, https://doi.org/10.1007/s13280-022-01723-1. . A 2022 report from thirty three experts highlights the need for “genuine participation by communities, inclusive project governance, integration of local work into national policies and practices, sustaining livelihoods and income (for example through the voluntary carbon market and/or national Payment for Ecosystem Services and other types of financial compensation systems) and simplification of carbon accounting and verification methodologies to lower barriers to entry[8]Dencer-Brown, Amrit Melissa, et al. “Integrating Blue: How Do We Make Nationally Determined Contributions Work for Both Blue Carbon and Local Coastal Communities?” Ambio, vol. 51, no. 9, 2022, pp. 1978–1993, https://doi.org/10.1007/s13280-022-01723-1. ” in order for blue carbon projects to be responsibly and successfully integrated into Nationally Determined Contributions.
- New efforts to improve the quality of carbon credits and the voluntary carbon market are being discussed and developed[9]https://blogs.edf.org/climate411/2023/08/01/navigating-the-core-carbon-principles-and-the-landscape-of-guidance-toward-a-high-integrity-carbon-market/ . The integrity of each carbon credit will be critical to enable the carbon market to address climate change. New guidance has been released in 2023 to ensure carbon credits deliver on their claims. The Integrity Council for the Voluntary Carbon Market released its Core Carbon Principles[10]https://icvcm.org/the-core-carbon-principles/ that set a global threshold to define what a high-integrity carbon credit is.
Challenges
- Verification of the amount of carbon sequestered and its durability can be extremely challenging across coastal vegetated ecosystems but is necessary for effective (in terms of mitigating climate change) carbon markets.
- Ensuring fairness and equity in benefits distribution
- Open systems where the open ocean is directly manipulated to enhance biological production, capture atmospheric CO2, and export the captured carbon to the deep ocean. In open systems, CO2 fixation is facilitated by the addition of limiting macronutrients (e.g., phosphorus, nitrogen, silica) and/or micronutrients (e.g., iron) to the ocean’s surface to augment biological production . Open system techniques accelerate natural processes already occurring in the ocean. Most approaches in the open ocean fall into the following two categories (some proposals that do not fit into these categories are also explored).
- Surface nutrient addition: the direct addition of nutrients (macro or micro) into ocean waters in situ to increase microalgal growth
- Nutrient upwelling: artificial upwelling of nutrient-rich deep ocean waters to the surface to increase microalgal growth
- Closed systems where inputs and growth conditions are controlled, and outputs (microalgae) are harvested within the confines of a pond or a photobioreactor. In closed systems, CO2 fixation is facilitated by the mixing of required inputs (sunlight, nutrients, CO2, water) and the introduction of microalgae culture with the intention of reproduction and continuous fixation in a contained system . This can be accomplished on shore in cultivation ponds or photobioreactors, or afloat in photobioreactors either stationary or towed at sea .
-
- Onshore: encompasses more established methods of microalgae cultivation, including photobioreactors, cultivation ponds, and hybrid onshore configurations. In these systems, all inputs are tightly controlled and regulated, and outputs must be directly managed through either storage or utilization of byproducts. While cultivation techniques are well-established and show high technological readiness, storage and utilization pathways remain underdeveloped and scale is a major consideration. Social and environmental risks for closed onshore systems are easier to monitor and mitigate due to the controlled nature of the system.
- Offshore: includes floating photobioreactors (PBRs) that are incorporated into a floating platform which can be stationary or towed behind a ship. In these systems, cultivation occurs in the photobioreactor, inputs are regulated, and outputs can be actively managed or directed. While at sea, these photobioreactors operate much like their onshore counterparts to cultivate microalgae, however nutrients and energy are provided by the ocean water and wave action, respectively. After microalgae are cultivated, they can be sunk into the deep ocean for sequestration or hauled to shore to be used as biomass. This is an area that has garnered much attention in startup communities (see this Y Combinator request for startups), however, little is available about the technologies in the open-sourced or peer reviewed literature.
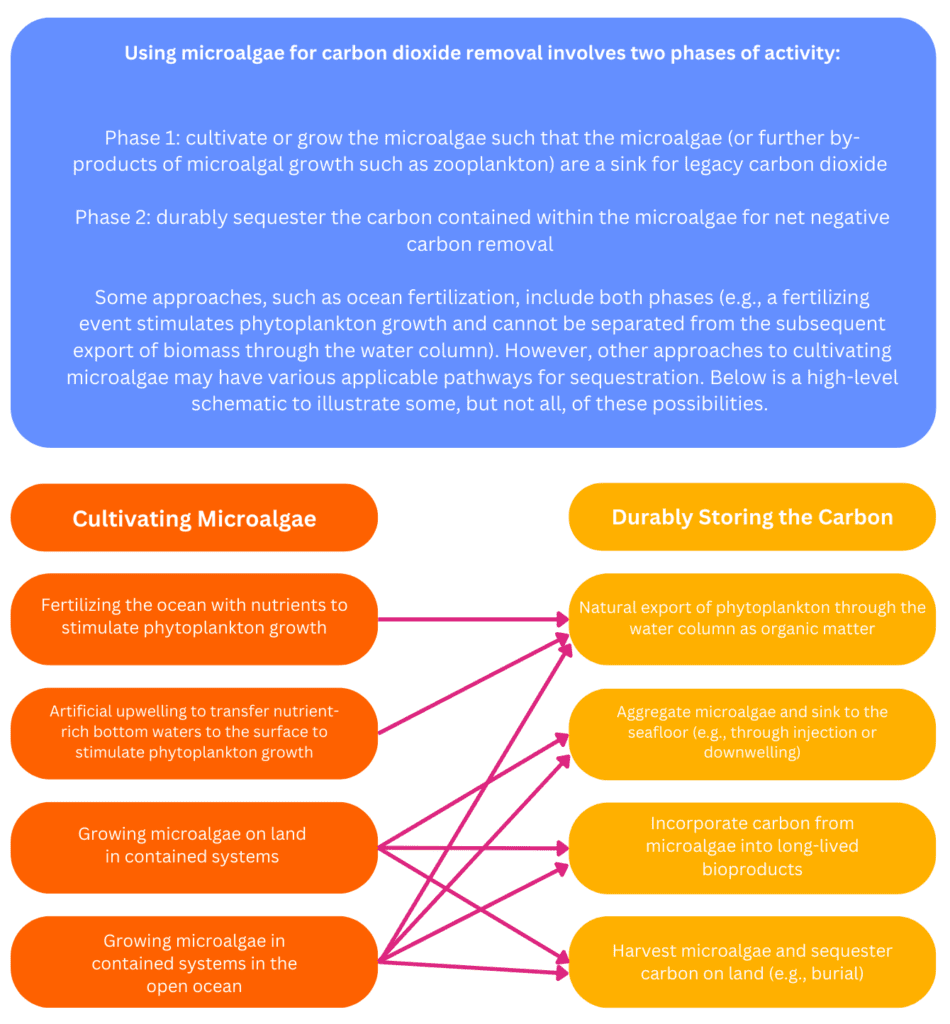
Ocean Visions. (2024) Ocean-Based Carbon Dioxide Removal: Road Maps. https://www2.oceanvisions.org/roadmaps/ remove/mcdr/ Accessed [insert date].
How to submit comments:
Thanks for helping to advance these roadmaps. Here is how it works!
Or, send general feedback to info@oceanvisions.org
All submitted comments will be reviewed by the Ocean Visions team to ensure that they are true, verifiable, and additive before being added to the road maps. You can increase the likelihood that your comments will be incorporated into the road maps by providing references (where appropriate).